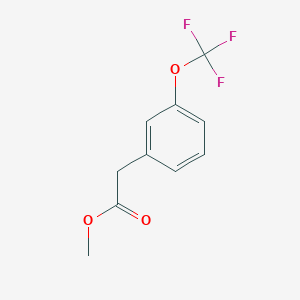
3-(Trifluoromethoxy)phenylacetic acid methyl ester
- Haga clic en CONSULTA RÁPIDA para recibir una cotización de nuestro equipo de expertos.
- Con productos de calidad a un precio COMPETITIVO, puede centrarse más en su investigación.
Descripción general
Descripción
3-(Trifluoromethoxy)phenylacetic acid methyl ester, also known as TFMPA methyl ester, is a chemical compound that has gained attention in the scientific community due to its potential applications in various fields.
Aplicaciones Científicas De Investigación
Photoreactivity and Electron Transfer
Research on substituted phenylacetic acid esters, including 1-naphthylmethyl esters, explores their photolysis reactions, revealing insights into radical and ion pair intermediates leading to various products. This study provides a foundation for understanding the photoreactivity and potential applications in photochemical syntheses involving similar ester compounds (DeCosta & Pincock, 1993).
Organic Solar Cells
Research on polymer/fullerene films, notably involving phenyl C61 butyric acid methyl ester derivatives, highlights their significance in improving the efficiency of organic solar cells through structural optimization. This application is crucial for the development of more efficient and sustainable energy sources (Erb et al., 2005).
Hyperbranched Polyimides
The synthesis of hyperbranched aromatic polyimides from monomers including tri(phthalic acid methyl ester) showcases the utility of esters in creating advanced polymer materials with potential applications in coatings, adhesives, and high-performance composites (Hao, Jikei, & Kakimoto, 2002).
Stereochemistry in Organic Synthesis
A study on the stereochemistry of 1,2,3-triols using ester derivatives provides insights into molecular conformations and stereochemical analysis, essential for the synthesis and characterization of chiral organic compounds (Freire et al., 2009).
Enhanced Photodynamic Therapy
The development of near-infrared photosensitizers for cancer therapy, incorporating ester derivatives, demonstrates the role of such compounds in medical applications, offering advancements in treatment options through targeted therapy and imaging (Patel et al., 2016).
Safety and Hazards
It is irritating to eyes and skin upon contact. Sulfuric acid, which is used in its synthesis, can cause chemical burns. Proper safety precautions should be followed when handling this compound .
Future Directions
Research on 3-(Trifluoromethoxy)phenylacetic acid methyl ester could explore its applications beyond derivatization, such as its potential use in organic synthesis or as a building block for more complex molecules. Investigating its reactivity with various nucleophiles and electrophiles could lead to novel synthetic methodologies .
Propiedades
IUPAC Name |
methyl 2-[3-(trifluoromethoxy)phenyl]acetate |
Source
|
---|---|---|
Details | Computed by LexiChem 2.6.6 (PubChem release 2019.06.18) | |
Source | PubChem | |
URL | https://pubchem.ncbi.nlm.nih.gov | |
Description | Data deposited in or computed by PubChem | |
InChI |
InChI=1S/C10H9F3O3/c1-15-9(14)6-7-3-2-4-8(5-7)16-10(11,12)13/h2-5H,6H2,1H3 |
Source
|
Details | Computed by InChI 1.0.5 (PubChem release 2019.06.18) | |
Source | PubChem | |
URL | https://pubchem.ncbi.nlm.nih.gov | |
Description | Data deposited in or computed by PubChem | |
InChI Key |
OINCZNUCGWDCGS-UHFFFAOYSA-N |
Source
|
Details | Computed by InChI 1.0.5 (PubChem release 2019.06.18) | |
Source | PubChem | |
URL | https://pubchem.ncbi.nlm.nih.gov | |
Description | Data deposited in or computed by PubChem | |
Canonical SMILES |
COC(=O)CC1=CC(=CC=C1)OC(F)(F)F |
Source
|
Details | Computed by OEChem 2.1.5 (PubChem release 2019.06.18) | |
Source | PubChem | |
URL | https://pubchem.ncbi.nlm.nih.gov | |
Description | Data deposited in or computed by PubChem | |
Molecular Formula |
C10H9F3O3 |
Source
|
Details | Computed by PubChem 2.1 (PubChem release 2019.06.18) | |
Source | PubChem | |
URL | https://pubchem.ncbi.nlm.nih.gov | |
Description | Data deposited in or computed by PubChem | |
Molecular Weight |
234.17 g/mol |
Source
|
Details | Computed by PubChem 2.1 (PubChem release 2021.05.07) | |
Source | PubChem | |
URL | https://pubchem.ncbi.nlm.nih.gov | |
Description | Data deposited in or computed by PubChem | |
Descargo de responsabilidad e información sobre productos de investigación in vitro
Tenga en cuenta que todos los artículos e información de productos presentados en BenchChem están destinados únicamente con fines informativos. Los productos disponibles para la compra en BenchChem están diseñados específicamente para estudios in vitro, que se realizan fuera de organismos vivos. Los estudios in vitro, derivados del término latino "in vidrio", involucran experimentos realizados en entornos de laboratorio controlados utilizando células o tejidos. Es importante tener en cuenta que estos productos no se clasifican como medicamentos y no han recibido la aprobación de la FDA para la prevención, tratamiento o cura de ninguna condición médica, dolencia o enfermedad. Debemos enfatizar que cualquier forma de introducción corporal de estos productos en humanos o animales está estrictamente prohibida por ley. Es esencial adherirse a estas pautas para garantizar el cumplimiento de los estándares legales y éticos en la investigación y experimentación.