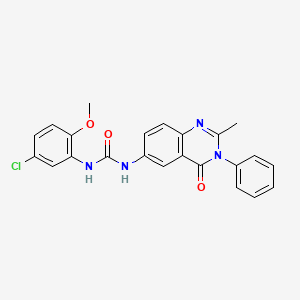
1-(5-Chloro-2-methoxyphenyl)-3-(2-methyl-4-oxo-3-phenyl-3,4-dihydroquinazolin-6-yl)urea
Descripción general
Descripción
1-(5-Chloro-2-methoxyphenyl)-3-(2-methyl-4-oxo-3-phenyl-3,4-dihydroquinazolin-6-yl)urea is a synthetic organic compound that belongs to the class of urea derivatives. This compound is characterized by its complex structure, which includes a quinazolinone moiety and a chloromethoxyphenyl group. It is of interest in various fields of scientific research due to its potential biological activities and applications in medicinal chemistry.
Métodos De Preparación
Synthetic Routes and Reaction Conditions
The synthesis of 1-(5-Chloro-2-methoxyphenyl)-3-(2-methyl-4-oxo-3-phenyl-3,4-dihydroquinazolin-6-yl)urea typically involves multiple steps:
Formation of the Quinazolinone Core: The quinazolinone core can be synthesized through the cyclization of anthranilic acid derivatives with appropriate aldehydes or ketones under acidic or basic conditions.
Introduction of the Chloromethoxyphenyl Group: The chloromethoxyphenyl group is introduced via nucleophilic substitution reactions, often using chlorinated aromatic compounds and methoxy-substituted phenols.
Urea Formation: The final step involves the reaction of the quinazolinone intermediate with an isocyanate or a carbamoyl chloride to form the urea linkage.
Industrial Production Methods
Industrial production of this compound would likely involve optimization of the above synthetic routes to ensure high yield and purity. This may include the use of continuous flow reactors, advanced purification techniques, and stringent quality control measures to meet industrial standards.
Análisis De Reacciones Químicas
Types of Reactions
1-(5-Chloro-2-methoxyphenyl)-3-(2-methyl-4-oxo-3-phenyl-3,4-dihydroquinazolin-6-yl)urea can undergo various chemical reactions, including:
Oxidation: The compound can be oxidized using strong oxidizing agents, leading to the formation of quinazolinone derivatives with different oxidation states.
Reduction: Reduction reactions can be performed using reducing agents like lithium aluminum hydride (LiAlH4) to yield reduced forms of the compound.
Common Reagents and Conditions
Oxidizing Agents: Potassium permanganate (KMnO4), chromium trioxide (CrO3).
Reducing Agents: Lithium aluminum hydride (LiAlH4), sodium borohydride (NaBH4).
Substitution Reagents: Sodium methoxide (NaOMe), various halides.
Major Products
The major products formed from these reactions depend on the specific conditions and reagents used. For example, oxidation may yield quinazolinone N-oxides, while reduction could produce dihydroquinazolinone derivatives.
Aplicaciones Científicas De Investigación
1-(5-Chloro-2-methoxyphenyl)-3-(2-methyl-4-oxo-3-phenyl-3,4-dihydroquinazolin-6-yl)urea has several scientific research applications:
Medicinal Chemistry: It is investigated for its potential as an anticancer agent due to its ability to inhibit certain enzymes and pathways involved in cancer cell proliferation.
Biological Studies: The compound is used in studies exploring its effects on various biological targets, including enzymes and receptors.
Industrial Applications: It may be used as an intermediate in the synthesis of other complex organic molecules with potential pharmaceutical applications.
Mecanismo De Acción
The mechanism of action of 1-(5-Chloro-2-methoxyphenyl)-3-(2-methyl-4-oxo-3-phenyl-3,4-dihydroquinazolin-6-yl)urea involves its interaction with specific molecular targets, such as enzymes or receptors. The compound may inhibit enzyme activity by binding to the active site or allosteric sites, thereby blocking substrate access or altering enzyme conformation. This inhibition can disrupt critical biological pathways, leading to the desired therapeutic effects.
Comparación Con Compuestos Similares
Similar Compounds
1-(5-Chloro-2-methoxyphenyl)-3-(2-methyl-4-oxo-3-phenyl-3,4-dihydroquinazolin-6-yl)thiourea: Similar structure but with a thiourea group instead of a urea group.
1-(5-Chloro-2-methoxyphenyl)-3-(2-methyl-4-oxo-3-phenyl-3,4-dihydroquinazolin-6-yl)carbamate: Contains a carbamate group instead of a urea group.
Uniqueness
The uniqueness of 1-(5-Chloro-2-methoxyphenyl)-3-(2-methyl-4-oxo-3-phenyl-3,4-dihydroquinazolin-6-yl)urea lies in its specific combination of functional groups, which confer distinct chemical and biological properties. Its structure allows for specific interactions with biological targets, making it a valuable compound for research and potential therapeutic applications.
Actividad Biológica
1-(5-Chloro-2-methoxyphenyl)-3-(2-methyl-4-oxo-3-phenyl-3,4-dihydroquinazolin-6-yl)urea is a synthetic compound with potential pharmacological applications. This article reviews its biological activities based on recent studies, including its effects on various biological targets, mechanisms of action, and potential therapeutic uses.
- Molecular Formula : C23H19ClN4O3
- Molecular Weight : 434.9 g/mol
- CAS Number : 1172784-73-4
Antitumor Activity
Research indicates that compounds similar to this compound exhibit significant antitumor properties. In particular, derivatives of this compound have shown promising results against various cancer cell lines. For example, a related compound demonstrated GI50 values (the concentration required to inhibit cell growth by 50%) of 25.1 µM against non-small cell lung cancer (EKVX) and lower values against leukemia and breast cancer cell lines .
Cell Line | GI50 (µM) |
---|---|
EKVX (Lung Cancer) | 25.1 |
RPMI-8226 (Leukemia) | 21.5 |
OVCAR-4 (Ovarian) | 25.9 |
MDA-MB-435 (Breast) | 15.1 |
Antimicrobial Activity
The compound has also been evaluated for its antimicrobial properties. Studies have shown that derivatives exhibit activity against both Gram-positive and Gram-negative bacteria. For instance, compounds in this class demonstrated minimum inhibitory concentrations (MICs) ranging from 0.3 to 8.5 µM against strains like Escherichia coli and Pseudomonas aeruginosa, indicating potential as antibacterial agents .
Bacterial Strain | MIC (µM) |
---|---|
E. coli | 0.3 - 8.5 |
S. aureus | 0.1 - 9.5 |
Pseudomonas aeruginosa | 0.3 - 8.5 |
Anti-inflammatory Activity
Another important aspect of the biological activity of this compound is its anti-inflammatory potential. Research has shown that certain urea derivatives can exhibit greater anti-inflammatory effects than curcumin, a well-known anti-inflammatory agent . This could position them as candidates for treating inflammatory diseases.
The mechanisms underlying the biological activities of this compound are still being elucidated. However, studies suggest that it may act through the inhibition of specific enzymes involved in cancer proliferation and inflammation pathways, such as GSK-3β . The structure–activity relationship (SAR) analyses indicate that modifications in the molecular structure can significantly impact the potency and selectivity of these compounds against various biological targets.
Case Studies
- Antitumor Efficacy : A recent study synthesized a series of urea derivatives, including those based on the quinazoline scaffold, which showed selective cytotoxicity towards cancer cells while sparing normal cells . This highlights the therapeutic potential of modifying the urea structure for enhanced efficacy.
- Antimicrobial Screening : In another study, a library of compounds was screened for antimicrobial activity against multiple bacterial strains, revealing that certain substitutions increased activity against resistant strains . This suggests that further optimization could yield more effective antimicrobial agents.
Q & A
Q. Basic: What are the optimized synthetic routes for this compound, and how do reaction conditions influence yield?
The synthesis typically involves multi-step reactions starting from substituted aniline derivatives. A common approach includes:
- Chlorination and functionalization of the methoxyphenyl group (e.g., using thionyl chloride or N-chlorosuccinimide) to introduce the 5-chloro-2-methoxy moiety.
- Formation of the quinazolinone core via cyclization of anthranilic acid derivatives with phenyl isocyanate or urea under acidic conditions.
- Coupling the urea linker using carbodiimide-mediated reactions (e.g., EDC/HOBt) to connect the chloromethoxyphenyl and quinazolinone fragments.
Critical factors for yield optimization :
- Temperature control : Excess heat during cyclization can lead to byproducts like dehalogenated intermediates.
- Solvent selection : Polar aprotic solvents (DMF, DMSO) enhance solubility of intermediates, while dichloromethane minimizes side reactions .
- Catalyst use : Palladium catalysts improve coupling efficiency in Suzuki-Miyaura reactions for aryl substitutions .
Q. Basic: What analytical methods are recommended for structural characterization and purity assessment?
A combination of spectroscopic and chromatographic techniques is essential:
- Nuclear Magnetic Resonance (NMR) : H and C NMR confirm the urea linkage (δ ~6.5–7.5 ppm for NH protons) and aromatic substituents.
- High-Resolution Mass Spectrometry (HRMS) : Validates molecular weight (e.g., calculated for CHClNO: 450.11 g/mol).
- High-Performance Liquid Chromatography (HPLC) : Reverse-phase C18 columns with UV detection at 254 nm assess purity (>95% required for biological assays) .
- X-ray crystallography : Resolves stereochemistry of the dihydroquinazolinone ring, critical for understanding binding interactions .
Q. Advanced: How can researchers resolve discrepancies between in vitro enzymatic inhibition and in vivo efficacy data?
Discrepancies often arise from pharmacokinetic limitations or off-target effects. Methodological strategies include:
- Metabolic stability assays : Use liver microsomes to identify rapid degradation (e.g., cytochrome P450-mediated oxidation of the methoxy group).
- Plasma protein binding studies : Equilibrium dialysis quantifies free drug availability.
- Pharmacophore modeling : Overlay the compound’s structure with known inhibitors to identify mismatched functional groups (e.g., steric clashes in the quinazolinone binding pocket) .
- Proteomics profiling : SILAC-based screens detect off-target interactions with unrelated kinases or receptors .
Q. Advanced: What structure-activity relationship (SAR) insights guide the design of analogs with improved selectivity?
Key SAR findings from analogous urea-quinazolinone derivatives:
Modification | Impact on Activity | Reference |
---|---|---|
Chloro → Fluoro substitution | Reduces off-target binding to hERG channels but decreases solubility. | |
Methoxy group removal | Enhances metabolic stability but reduces affinity for hydrophobic binding pockets. | |
Quinazolinone N-methylation | Improves blood-brain barrier penetration but increases cytotoxicity in hepatic cells. |
Design recommendations :
- Introduce electron-withdrawing groups (e.g., trifluoromethyl) on the phenyl ring to balance solubility and target affinity.
- Replace the urea linker with thiourea or carbamate to modulate hydrogen-bonding interactions .
Q. Advanced: How should researchers validate target engagement in complex biological systems?
Use orthogonal assays to confirm mechanism of action:
- Cellular thermal shift assay (CETSA) : Monitor target protein stabilization upon compound binding in lysates or live cells.
- Surface plasmon resonance (SPR) : Measure real-time binding kinetics (e.g., K < 100 nM for kinase targets).
- Knockdown/rescue experiments : siRNA-mediated target silencing followed by compound treatment confirms specificity (e.g., rescue via overexpression of wild-type but not mutant protein) .
Q. Advanced: What strategies mitigate toxicity observed in preliminary cytotoxicity screens?
- Reactive metabolite screening : Glutathione trapping assays identify thiol-reactive intermediates formed via metabolic activation.
- Chelation of metal ions : Test if toxicity arises from unintended interactions with zinc or iron cofactors.
- Prodrug approaches : Mask polar groups (e.g., esterify the urea NH) to reduce off-target accumulation .
Q. Advanced: How can computational methods enhance the understanding of binding dynamics?
- Molecular dynamics (MD) simulations : Simulate ligand-protein interactions over 100+ ns to identify stable binding poses (e.g., quinazolinone stacking with tyrosine residues).
- Free energy perturbation (FEP) : Predict ΔΔG for substituent modifications with <1 kcal/mol error margins.
- Density functional theory (DFT) : Calculate electrostatic potential maps to optimize charge distribution .
Propiedades
IUPAC Name |
1-(5-chloro-2-methoxyphenyl)-3-(2-methyl-4-oxo-3-phenylquinazolin-6-yl)urea | |
---|---|---|
Details | Computed by Lexichem TK 2.7.0 (PubChem release 2021.05.07) | |
Source | PubChem | |
URL | https://pubchem.ncbi.nlm.nih.gov | |
Description | Data deposited in or computed by PubChem | |
InChI |
InChI=1S/C23H19ClN4O3/c1-14-25-19-10-9-16(13-18(19)22(29)28(14)17-6-4-3-5-7-17)26-23(30)27-20-12-15(24)8-11-21(20)31-2/h3-13H,1-2H3,(H2,26,27,30) | |
Details | Computed by InChI 1.0.6 (PubChem release 2021.05.07) | |
Source | PubChem | |
URL | https://pubchem.ncbi.nlm.nih.gov | |
Description | Data deposited in or computed by PubChem | |
InChI Key |
XCIKRZPIVSCZHJ-UHFFFAOYSA-N | |
Details | Computed by InChI 1.0.6 (PubChem release 2021.05.07) | |
Source | PubChem | |
URL | https://pubchem.ncbi.nlm.nih.gov | |
Description | Data deposited in or computed by PubChem | |
Canonical SMILES |
CC1=NC2=C(C=C(C=C2)NC(=O)NC3=C(C=CC(=C3)Cl)OC)C(=O)N1C4=CC=CC=C4 | |
Details | Computed by OEChem 2.3.0 (PubChem release 2021.05.07) | |
Source | PubChem | |
URL | https://pubchem.ncbi.nlm.nih.gov | |
Description | Data deposited in or computed by PubChem | |
Molecular Formula |
C23H19ClN4O3 | |
Details | Computed by PubChem 2.1 (PubChem release 2021.05.07) | |
Source | PubChem | |
URL | https://pubchem.ncbi.nlm.nih.gov | |
Description | Data deposited in or computed by PubChem | |
Molecular Weight |
434.9 g/mol | |
Details | Computed by PubChem 2.1 (PubChem release 2021.05.07) | |
Source | PubChem | |
URL | https://pubchem.ncbi.nlm.nih.gov | |
Description | Data deposited in or computed by PubChem | |
Descargo de responsabilidad e información sobre productos de investigación in vitro
Tenga en cuenta que todos los artículos e información de productos presentados en BenchChem están destinados únicamente con fines informativos. Los productos disponibles para la compra en BenchChem están diseñados específicamente para estudios in vitro, que se realizan fuera de organismos vivos. Los estudios in vitro, derivados del término latino "in vidrio", involucran experimentos realizados en entornos de laboratorio controlados utilizando células o tejidos. Es importante tener en cuenta que estos productos no se clasifican como medicamentos y no han recibido la aprobación de la FDA para la prevención, tratamiento o cura de ninguna condición médica, dolencia o enfermedad. Debemos enfatizar que cualquier forma de introducción corporal de estos productos en humanos o animales está estrictamente prohibida por ley. Es esencial adherirse a estas pautas para garantizar el cumplimiento de los estándares legales y éticos en la investigación y experimentación.