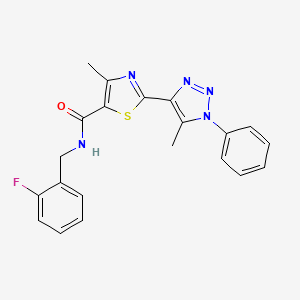
N-(2-fluorobenzyl)-4-methyl-2-(5-methyl-1-phenyl-1H-1,2,3-triazol-4-yl)thiazole-5-carboxamide
- Haga clic en CONSULTA RÁPIDA para recibir una cotización de nuestro equipo de expertos.
- Con productos de calidad a un precio COMPETITIVO, puede centrarse más en su investigación.
Descripción general
Descripción
N-(2-fluorobenzyl)-4-methyl-2-(5-methyl-1-phenyl-1H-1,2,3-triazol-4-yl)thiazole-5-carboxamide is a useful research compound. Its molecular formula is C21H18FN5OS and its molecular weight is 407.47. The purity is usually 95%.
BenchChem offers high-quality N-(2-fluorobenzyl)-4-methyl-2-(5-methyl-1-phenyl-1H-1,2,3-triazol-4-yl)thiazole-5-carboxamide suitable for many research applications. Different packaging options are available to accommodate customers' requirements. Please inquire for more information about N-(2-fluorobenzyl)-4-methyl-2-(5-methyl-1-phenyl-1H-1,2,3-triazol-4-yl)thiazole-5-carboxamide including the price, delivery time, and more detailed information at info@benchchem.com.
Aplicaciones Científicas De Investigación
Synthesis and Chemical Properties
- Research on thiazole derivatives, including the synthesis of 2-substituted thiazole-5-carboxylates, demonstrates the versatility of thiazole compounds in organic synthesis. These studies often explore novel synthetic routes and the chemical reactivity of thiazole derivatives, providing a basis for developing new compounds with potential research and therapeutic applications (Fong et al., 2004).
Antibacterial and Antituberculosis Activity
- Thiazole and triazole hybrids have shown promise as antibacterial and antituberculosis agents. For instance, a study on thiazole-aminopiperidine hybrid analogues revealed their effectiveness as Mycobacterium tuberculosis GyrB inhibitors, highlighting the potential of thiazole derivatives in addressing infectious diseases (Jeankumar et al., 2013).
Anticancer Activity
- Fluorinated benzothiazoles have been studied for their in vitro cytotoxic properties against various cancer cell lines. Such research contributes to the development of new anticancer agents, exploring the role of fluorine substitution in modulating biological activity (Hutchinson et al., 2001).
Antimicrobial Activity
- The synthesis and biological evaluation of thiazole derivatives have revealed significant antimicrobial activities, demonstrating the potential of these compounds in developing new antimicrobial agents. Research in this area often focuses on identifying compounds with effective antibacterial and antifungal properties (Mhaske et al., 2011).
Propiedades
IUPAC Name |
N-[(2-fluorophenyl)methyl]-4-methyl-2-(5-methyl-1-phenyltriazol-4-yl)-1,3-thiazole-5-carboxamide |
Source
|
---|---|---|
Details | Computed by Lexichem TK 2.7.0 (PubChem release 2021.05.07) | |
Source | PubChem | |
URL | https://pubchem.ncbi.nlm.nih.gov | |
Description | Data deposited in or computed by PubChem | |
InChI |
InChI=1S/C21H18FN5OS/c1-13-19(20(28)23-12-15-8-6-7-11-17(15)22)29-21(24-13)18-14(2)27(26-25-18)16-9-4-3-5-10-16/h3-11H,12H2,1-2H3,(H,23,28) |
Source
|
Details | Computed by InChI 1.0.6 (PubChem release 2021.05.07) | |
Source | PubChem | |
URL | https://pubchem.ncbi.nlm.nih.gov | |
Description | Data deposited in or computed by PubChem | |
InChI Key |
XKJUXXZVGTURLC-UHFFFAOYSA-N |
Source
|
Details | Computed by InChI 1.0.6 (PubChem release 2021.05.07) | |
Source | PubChem | |
URL | https://pubchem.ncbi.nlm.nih.gov | |
Description | Data deposited in or computed by PubChem | |
Canonical SMILES |
CC1=C(SC(=N1)C2=C(N(N=N2)C3=CC=CC=C3)C)C(=O)NCC4=CC=CC=C4F |
Source
|
Details | Computed by OEChem 2.3.0 (PubChem release 2021.05.07) | |
Source | PubChem | |
URL | https://pubchem.ncbi.nlm.nih.gov | |
Description | Data deposited in or computed by PubChem | |
Molecular Formula |
C21H18FN5OS |
Source
|
Details | Computed by PubChem 2.1 (PubChem release 2021.05.07) | |
Source | PubChem | |
URL | https://pubchem.ncbi.nlm.nih.gov | |
Description | Data deposited in or computed by PubChem | |
Molecular Weight |
407.5 g/mol |
Source
|
Details | Computed by PubChem 2.1 (PubChem release 2021.05.07) | |
Source | PubChem | |
URL | https://pubchem.ncbi.nlm.nih.gov | |
Description | Data deposited in or computed by PubChem | |
Product Name |
N-(2-fluorobenzyl)-4-methyl-2-(5-methyl-1-phenyl-1H-1,2,3-triazol-4-yl)thiazole-5-carboxamide |
Descargo de responsabilidad e información sobre productos de investigación in vitro
Tenga en cuenta que todos los artículos e información de productos presentados en BenchChem están destinados únicamente con fines informativos. Los productos disponibles para la compra en BenchChem están diseñados específicamente para estudios in vitro, que se realizan fuera de organismos vivos. Los estudios in vitro, derivados del término latino "in vidrio", involucran experimentos realizados en entornos de laboratorio controlados utilizando células o tejidos. Es importante tener en cuenta que estos productos no se clasifican como medicamentos y no han recibido la aprobación de la FDA para la prevención, tratamiento o cura de ninguna condición médica, dolencia o enfermedad. Debemos enfatizar que cualquier forma de introducción corporal de estos productos en humanos o animales está estrictamente prohibida por ley. Es esencial adherirse a estas pautas para garantizar el cumplimiento de los estándares legales y éticos en la investigación y experimentación.