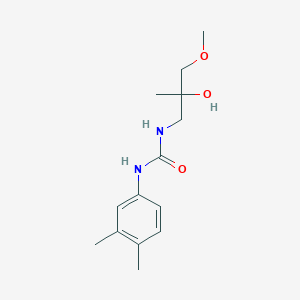
1-(3,4-Dimethylphenyl)-3-(2-hydroxy-3-methoxy-2-methylpropyl)urea
- Haga clic en CONSULTA RÁPIDA para recibir una cotización de nuestro equipo de expertos.
- Con productos de calidad a un precio COMPETITIVO, puede centrarse más en su investigación.
Descripción general
Descripción
1-(3,4-Dimethylphenyl)-3-(2-hydroxy-3-methoxy-2-methylpropyl)urea, also known as DMHU, is a chemical compound that has been of great interest to the scientific community due to its potential applications in various fields, including medicine, agriculture, and environmental science. DMHU belongs to the class of urea derivatives and has been synthesized using various methods.
Aplicaciones Científicas De Investigación
Environmental Applications
Urea derivatives are extensively studied for their environmental behavior, particularly in the context of herbicides and pollutants. For instance, urea-based herbicides such as isoproturon are monitored in environmental matrices due to their widespread use and potential for contamination. The development of sensitive detection methods, such as enzyme-linked immunosorbent assays (ELISAs), facilitates monitoring their presence in water and soil, ensuring the protection of ecosystems and human health (Katmeh et al., 1994). Furthermore, the identification of bacterial strains capable of degrading phenylurea herbicides, such as isoproturon, highlights the importance of bioremediation strategies in mitigating environmental pollution (Sørensen et al., 2001).
Synthetic and Medicinal Chemistry
In synthetic chemistry, urea derivatives are key intermediates in the preparation of various compounds. Hindered ureas, for example, have been shown to undergo efficient substitution reactions with a range of nucleophiles under neutral conditions, highlighting their versatility in organic synthesis (Hutchby et al., 2009). This property is leveraged in the development of novel therapeutic agents, where the structural motif of urea is incorporated into drug molecules to enhance their biological activity. A study on the synthesis and biological evaluation of 1-aryl-3-[4-(pyridin-2-ylmethoxy)phenyl]urea derivatives as anticancer agents exemplifies the ongoing research in utilizing urea derivatives for medicinal purposes (Feng et al., 2020).
Material Science
In material science, urea-formaldehyde resins are widely used due to their mechanical strength, chemical resistance, and adhesive properties. Detailed studies using various spectroscopic methods, including 1H, 13C, and 15N NMR, have shed light on the complex reaction networks involved in their synthesis, providing insights into optimizing their properties for specific applications (Steinhof et al., 2014).
Propiedades
IUPAC Name |
1-(3,4-dimethylphenyl)-3-(2-hydroxy-3-methoxy-2-methylpropyl)urea |
Source
|
---|---|---|
Details | Computed by LexiChem 2.6.6 (PubChem release 2019.06.18) | |
Source | PubChem | |
URL | https://pubchem.ncbi.nlm.nih.gov | |
Description | Data deposited in or computed by PubChem | |
InChI |
InChI=1S/C14H22N2O3/c1-10-5-6-12(7-11(10)2)16-13(17)15-8-14(3,18)9-19-4/h5-7,18H,8-9H2,1-4H3,(H2,15,16,17) |
Source
|
Details | Computed by InChI 1.0.5 (PubChem release 2019.06.18) | |
Source | PubChem | |
URL | https://pubchem.ncbi.nlm.nih.gov | |
Description | Data deposited in or computed by PubChem | |
InChI Key |
GATHQSCTVXFGFC-UHFFFAOYSA-N |
Source
|
Details | Computed by InChI 1.0.5 (PubChem release 2019.06.18) | |
Source | PubChem | |
URL | https://pubchem.ncbi.nlm.nih.gov | |
Description | Data deposited in or computed by PubChem | |
Canonical SMILES |
CC1=C(C=C(C=C1)NC(=O)NCC(C)(COC)O)C |
Source
|
Details | Computed by OEChem 2.1.5 (PubChem release 2019.06.18) | |
Source | PubChem | |
URL | https://pubchem.ncbi.nlm.nih.gov | |
Description | Data deposited in or computed by PubChem | |
Molecular Formula |
C14H22N2O3 |
Source
|
Details | Computed by PubChem 2.1 (PubChem release 2019.06.18) | |
Source | PubChem | |
URL | https://pubchem.ncbi.nlm.nih.gov | |
Description | Data deposited in or computed by PubChem | |
Molecular Weight |
266.34 g/mol |
Source
|
Details | Computed by PubChem 2.1 (PubChem release 2021.05.07) | |
Source | PubChem | |
URL | https://pubchem.ncbi.nlm.nih.gov | |
Description | Data deposited in or computed by PubChem | |
Product Name |
1-(3,4-Dimethylphenyl)-3-(2-hydroxy-3-methoxy-2-methylpropyl)urea |
Descargo de responsabilidad e información sobre productos de investigación in vitro
Tenga en cuenta que todos los artículos e información de productos presentados en BenchChem están destinados únicamente con fines informativos. Los productos disponibles para la compra en BenchChem están diseñados específicamente para estudios in vitro, que se realizan fuera de organismos vivos. Los estudios in vitro, derivados del término latino "in vidrio", involucran experimentos realizados en entornos de laboratorio controlados utilizando células o tejidos. Es importante tener en cuenta que estos productos no se clasifican como medicamentos y no han recibido la aprobación de la FDA para la prevención, tratamiento o cura de ninguna condición médica, dolencia o enfermedad. Debemos enfatizar que cualquier forma de introducción corporal de estos productos en humanos o animales está estrictamente prohibida por ley. Es esencial adherirse a estas pautas para garantizar el cumplimiento de los estándares legales y éticos en la investigación y experimentación.