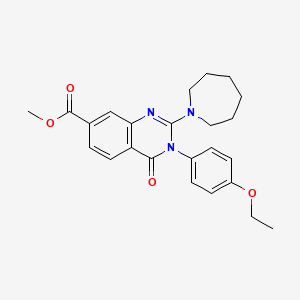
Methyl 2-(azepan-1-yl)-3-(4-ethoxyphenyl)-4-oxo-3,4-dihydroquinazoline-7-carboxylate
- Haga clic en CONSULTA RÁPIDA para recibir una cotización de nuestro equipo de expertos.
- Con productos de calidad a un precio COMPETITIVO, puede centrarse más en su investigación.
Descripción general
Descripción
Methyl 2-(azepan-1-yl)-3-(4-ethoxyphenyl)-4-oxo-3,4-dihydroquinazoline-7-carboxylate is a useful research compound. Its molecular formula is C24H27N3O4 and its molecular weight is 421.497. The purity is usually 95%.
BenchChem offers high-quality Methyl 2-(azepan-1-yl)-3-(4-ethoxyphenyl)-4-oxo-3,4-dihydroquinazoline-7-carboxylate suitable for many research applications. Different packaging options are available to accommodate customers' requirements. Please inquire for more information about Methyl 2-(azepan-1-yl)-3-(4-ethoxyphenyl)-4-oxo-3,4-dihydroquinazoline-7-carboxylate including the price, delivery time, and more detailed information at info@benchchem.com.
Aplicaciones Científicas De Investigación
Cyclization and Synthesis Applications
Cyclization of Amino-Acid Derivatives : Research into the cyclization of amino-acid derivatives led to the synthesis of compounds such as methyl 1,2,3,4-tetrahydro-4-oxoquinoline-3-carboxylate, showcasing methods for creating tetrahydroquinolin-4-ones and tetrahydro-1-benzazepin-5-ones through Dieckmann cyclisation (Proctor, Ross, & Tapia, 1972).
Synthesis of Azuleno Derivatives : The synthesis of new heterocyclic compounds, including methyl 1,2-dihydroazuleno[1,2-b]pyrrole-9-carboxylate, demonstrates the potential for creating novel structures with potential pharmaceutical relevance (Fujimori et al., 1986).
Palladium-Catalyzed Oxidative Cyclization-Alkoxycarbonylation : This method was used to synthesize various heterocyclic derivatives, including tetrahydrofuran, dioxolane, oxazoline, dihydropyridinone, and tetrahydropyridinedione derivatives, showcasing the versatility of palladium-catalyzed reactions in synthesizing complex structures (Bacchi et al., 2005).
Structural and Mechanistic Studies
Hydroformylation of Ortho-Propenylanilines : This study explored the Rhodium-catalyzed hydroformylation of ortho-propenylanilines, leading to the synthesis of 4,5-dihydro-3H-1-benzazepines, highlighting a novel route to this class of compounds (Anastasiou & Jackson, 1992).
Synthesis of 1,3,4-Benzotriazepines : Demonstrating the reaction between 2-(2′,2′-bis-carboethoxyvinylamino)-benzophenones and hydrazine hydrate, this study shows a method for synthesizing 5-hydroxy-5-phenyl-1,3,4-3H-4,5-dihydrobenzotriazepines, offering insights into the structural versatility of benzotriazepines (Podesva, Kohan, & Vagi, 1969).
Propiedades
IUPAC Name |
methyl 2-(azepan-1-yl)-3-(4-ethoxyphenyl)-4-oxoquinazoline-7-carboxylate |
Source
|
---|---|---|
Details | Computed by Lexichem TK 2.7.0 (PubChem release 2021.05.07) | |
Source | PubChem | |
URL | https://pubchem.ncbi.nlm.nih.gov | |
Description | Data deposited in or computed by PubChem | |
InChI |
InChI=1S/C24H27N3O4/c1-3-31-19-11-9-18(10-12-19)27-22(28)20-13-8-17(23(29)30-2)16-21(20)25-24(27)26-14-6-4-5-7-15-26/h8-13,16H,3-7,14-15H2,1-2H3 |
Source
|
Details | Computed by InChI 1.0.6 (PubChem release 2021.05.07) | |
Source | PubChem | |
URL | https://pubchem.ncbi.nlm.nih.gov | |
Description | Data deposited in or computed by PubChem | |
InChI Key |
ONTDFOFMXWPINI-UHFFFAOYSA-N |
Source
|
Details | Computed by InChI 1.0.6 (PubChem release 2021.05.07) | |
Source | PubChem | |
URL | https://pubchem.ncbi.nlm.nih.gov | |
Description | Data deposited in or computed by PubChem | |
Canonical SMILES |
CCOC1=CC=C(C=C1)N2C(=O)C3=C(C=C(C=C3)C(=O)OC)N=C2N4CCCCCC4 |
Source
|
Details | Computed by OEChem 2.3.0 (PubChem release 2021.05.07) | |
Source | PubChem | |
URL | https://pubchem.ncbi.nlm.nih.gov | |
Description | Data deposited in or computed by PubChem | |
Molecular Formula |
C24H27N3O4 |
Source
|
Details | Computed by PubChem 2.1 (PubChem release 2021.05.07) | |
Source | PubChem | |
URL | https://pubchem.ncbi.nlm.nih.gov | |
Description | Data deposited in or computed by PubChem | |
Molecular Weight |
421.5 g/mol |
Source
|
Details | Computed by PubChem 2.1 (PubChem release 2021.05.07) | |
Source | PubChem | |
URL | https://pubchem.ncbi.nlm.nih.gov | |
Description | Data deposited in or computed by PubChem | |
Product Name |
Methyl 2-(azepan-1-yl)-3-(4-ethoxyphenyl)-4-oxo-3,4-dihydroquinazoline-7-carboxylate |
Descargo de responsabilidad e información sobre productos de investigación in vitro
Tenga en cuenta que todos los artículos e información de productos presentados en BenchChem están destinados únicamente con fines informativos. Los productos disponibles para la compra en BenchChem están diseñados específicamente para estudios in vitro, que se realizan fuera de organismos vivos. Los estudios in vitro, derivados del término latino "in vidrio", involucran experimentos realizados en entornos de laboratorio controlados utilizando células o tejidos. Es importante tener en cuenta que estos productos no se clasifican como medicamentos y no han recibido la aprobación de la FDA para la prevención, tratamiento o cura de ninguna condición médica, dolencia o enfermedad. Debemos enfatizar que cualquier forma de introducción corporal de estos productos en humanos o animales está estrictamente prohibida por ley. Es esencial adherirse a estas pautas para garantizar el cumplimiento de los estándares legales y éticos en la investigación y experimentación.