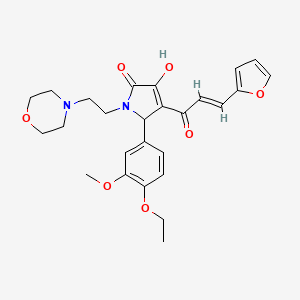
(E)-5-(4-ethoxy-3-methoxyphenyl)-4-(3-(furan-2-yl)acryloyl)-3-hydroxy-1-(2-morpholinoethyl)-1H-pyrrol-2(5H)-one
- Haga clic en CONSULTA RÁPIDA para recibir una cotización de nuestro equipo de expertos.
- Con productos de calidad a un precio COMPETITIVO, puede centrarse más en su investigación.
Descripción general
Descripción
(E)-5-(4-ethoxy-3-methoxyphenyl)-4-(3-(furan-2-yl)acryloyl)-3-hydroxy-1-(2-morpholinoethyl)-1H-pyrrol-2(5H)-one is a useful research compound. Its molecular formula is C26H30N2O7 and its molecular weight is 482.533. The purity is usually 95%.
BenchChem offers high-quality this compound suitable for many research applications. Different packaging options are available to accommodate customers' requirements. Please inquire for more information about this compound including the price, delivery time, and more detailed information at info@benchchem.com.
Aplicaciones Científicas De Investigación
Synthesis and Characterization
- Pyrrole chalcone derivatives, such as ethyl 4-(3-Furan-2yl-acryloyl)-3,5-dimethyl-1H-pyrrole-2-carboxylate, have been synthesized through aldol condensation, highlighting the utility of pyrrole derivatives in forming heterocyclic compounds like oxiranes, oxazoles, pyrazoles, and pyridines. These processes are essential for developing materials with potential applications in pharmaceuticals and polymers (R. N. Singh, Poonam Rawat, S. Sahu, 2014).
Computational Study
- Quantum chemical calculations alongside experimental data have been used to study the molecular electrostatic potential, electronic descriptors, and natural bond orbital interactions of pyrrole derivatives. Such computational studies aid in predicting interaction sites and the nature of these interactions, providing insights into the molecule's reactivity and potential applications in designing new compounds (R. N. Singh, Poonam Rawat, S. Sahu, 2014).
Metal-Free Synthesis
- An efficient, metal-free method for synthesizing polysubstituted pyrrole derivatives has been developed, showcasing the environmental benefits of such processes. This research is particularly relevant for green chemistry, emphasizing the importance of sustainable methods in chemical synthesis (Amrendra Kumar, Rāmānand, N. Tadigoppula, 2017).
Biological Evaluation
- Pyrrole derivatives have been studied for their biological activities, including anti-inflammatory and antibacterial properties. This research underlines the potential of pyrrole-based compounds in medicinal chemistry and drug design, highlighting their relevance in developing new therapeutic agents (P. Ravula, V. Babu, P. Manich, Rika, N. R. Chary, J. N. S. Ch, Ra, 2016).
Propiedades
IUPAC Name |
2-(4-ethoxy-3-methoxyphenyl)-3-[(E)-3-(furan-2-yl)prop-2-enoyl]-4-hydroxy-1-(2-morpholin-4-ylethyl)-2H-pyrrol-5-one |
Source
|
---|---|---|
Details | Computed by Lexichem TK 2.7.0 (PubChem release 2021.05.07) | |
Source | PubChem | |
URL | https://pubchem.ncbi.nlm.nih.gov | |
Description | Data deposited in or computed by PubChem | |
InChI |
InChI=1S/C26H30N2O7/c1-3-34-21-9-6-18(17-22(21)32-2)24-23(20(29)8-7-19-5-4-14-35-19)25(30)26(31)28(24)11-10-27-12-15-33-16-13-27/h4-9,14,17,24,30H,3,10-13,15-16H2,1-2H3/b8-7+ |
Source
|
Details | Computed by InChI 1.0.6 (PubChem release 2021.05.07) | |
Source | PubChem | |
URL | https://pubchem.ncbi.nlm.nih.gov | |
Description | Data deposited in or computed by PubChem | |
InChI Key |
WBGGXSFEZDUWQY-BQYQJAHWSA-N |
Source
|
Details | Computed by InChI 1.0.6 (PubChem release 2021.05.07) | |
Source | PubChem | |
URL | https://pubchem.ncbi.nlm.nih.gov | |
Description | Data deposited in or computed by PubChem | |
Canonical SMILES |
CCOC1=C(C=C(C=C1)C2C(=C(C(=O)N2CCN3CCOCC3)O)C(=O)C=CC4=CC=CO4)OC |
Source
|
Details | Computed by OEChem 2.3.0 (PubChem release 2021.05.07) | |
Source | PubChem | |
URL | https://pubchem.ncbi.nlm.nih.gov | |
Description | Data deposited in or computed by PubChem | |
Isomeric SMILES |
CCOC1=C(C=C(C=C1)C2C(=C(C(=O)N2CCN3CCOCC3)O)C(=O)/C=C/C4=CC=CO4)OC |
Source
|
Details | Computed by OEChem 2.3.0 (PubChem release 2021.05.07) | |
Source | PubChem | |
URL | https://pubchem.ncbi.nlm.nih.gov | |
Description | Data deposited in or computed by PubChem | |
Molecular Formula |
C26H30N2O7 |
Source
|
Details | Computed by PubChem 2.1 (PubChem release 2021.05.07) | |
Source | PubChem | |
URL | https://pubchem.ncbi.nlm.nih.gov | |
Description | Data deposited in or computed by PubChem | |
Molecular Weight |
482.5 g/mol |
Source
|
Details | Computed by PubChem 2.1 (PubChem release 2021.05.07) | |
Source | PubChem | |
URL | https://pubchem.ncbi.nlm.nih.gov | |
Description | Data deposited in or computed by PubChem | |
Descargo de responsabilidad e información sobre productos de investigación in vitro
Tenga en cuenta que todos los artículos e información de productos presentados en BenchChem están destinados únicamente con fines informativos. Los productos disponibles para la compra en BenchChem están diseñados específicamente para estudios in vitro, que se realizan fuera de organismos vivos. Los estudios in vitro, derivados del término latino "in vidrio", involucran experimentos realizados en entornos de laboratorio controlados utilizando células o tejidos. Es importante tener en cuenta que estos productos no se clasifican como medicamentos y no han recibido la aprobación de la FDA para la prevención, tratamiento o cura de ninguna condición médica, dolencia o enfermedad. Debemos enfatizar que cualquier forma de introducción corporal de estos productos en humanos o animales está estrictamente prohibida por ley. Es esencial adherirse a estas pautas para garantizar el cumplimiento de los estándares legales y éticos en la investigación y experimentación.