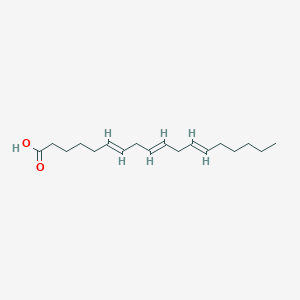
6,9,12-Octadecatrienoic acid
- Haga clic en CONSULTA RÁPIDA para recibir una cotización de nuestro equipo de expertos.
- Con productos de calidad a un precio COMPETITIVO, puede centrarse más en su investigación.
Descripción general
Descripción
6,9,12-Octadecatrienoic acid, commonly known as alpha-linolenic acid (ALA), is a polyunsaturated fatty acid that belongs to the omega-3 family. It is an essential fatty acid that cannot be synthesized by the human body and must be obtained through the diet. ALA is found in various plant sources, including flaxseed, chia seeds, hemp seeds, walnuts, and soybeans. In recent years, ALA has gained significant attention due to its potential health benefits, including its ability to reduce inflammation, improve heart health, and support brain function.
Aplicaciones Científicas De Investigación
Pheromone and Attractant Synthesis
6,9,12-Octadecatrienoic acid is utilized in synthesizing pheromone and attractant components for lepidopterous insect pests. Efficient chemical syntheses of these components have been reported using this acid (Wang & Zhang, 2007).
Bioactive Properties and Metabolism
This compound exhibits cardiovascular-protective, anti-cancer, neuro-protective, anti-inflammatory, and antioxidant properties. It's converted into eicosa-pentaenoic acid (EPA) and docosa-hexaenoic acid (DHA) in the human system, influencing the risk of ischemic heart disease and blood clots (Parvathi et al., 2022).
Biosynthesis Studies
Gamma-linolenic acid, a variant of this compound, is biosynthesized through a series of dehydrogenation reactions catalyzed by fatty acid desaturases. Studies on the cryptoregiochemistry of these reactions provide insights into the biosynthesis process (Fauconnot & Buist, 2001).
Fungal Desaturation Characteristics
Research on fungi like Trichoderma sp. explores how they introduce double bonds in fatty acids, including this compound, demonstrating the complexity of fungal lipid metabolism (Shirasaka et al., 2005).
Synthetic Chemistry
The synthesis of 3-oxalinolenic acid from this compound showcases the ability to create beta-oxidation-resistant oxylipins, which might have enhanced biological activity due to resistance to metabolic degradation (Hamberg et al., 2006).
Metabolism Studies
Synthetic approaches like the creation of radiolabeled analogs of octadeca-6,10,12-trienoic acid help in studying the metabolic fate of conjugated linoleic acid isomers, crucial for understanding its role in biological systems (Loreau et al., 2003).
Aromatase Inhibition
Compounds derived from this compound, isolated from various plants, have demonstrated strong aromatase inhibitory activity, highlighting its potential in medicinal chemistry (Yang et al., 2009).
Corrosion Inhibition
Fatty acids including this compound have shown potential in corrosion inhibition of metals, a novel application in materials science (Khanra et al., 2018).
Fungal Production of Polyunsaturated Fatty Acids
Microbial production of polyunsaturated fatty acids, including variants of this compound, has been extensively researched for potential applications in nutrition and pharmaceuticals (Arjuna, 2014).
Biofuel Properties
Studying the fuel properties of methyl esters containing this compound provides insights into the potential of these fatty acids in biofuel applications (Knothe, 2013).
Propiedades
Número CAS |
1686-12-0 |
---|---|
Fórmula molecular |
C18H30O2 |
Peso molecular |
278.4 g/mol |
Nombre IUPAC |
(6E,9E,12E)-octadeca-6,9,12-trienoic acid |
InChI |
InChI=1S/C18H30O2/c1-2-3-4-5-6-7-8-9-10-11-12-13-14-15-16-17-18(19)20/h6-7,9-10,12-13H,2-5,8,11,14-17H2,1H3,(H,19,20)/b7-6+,10-9+,13-12+ |
Clave InChI |
VZCCETWTMQHEPK-YHTMAJSVSA-N |
SMILES isomérico |
CCCCC/C=C/C/C=C/C/C=C/CCCCC(=O)O |
SMILES |
CCCCCC=CCC=CCC=CCCCCC(=O)O |
SMILES canónico |
CCCCCC=CCC=CCC=CCCCCC(=O)O |
Sinónimos |
LINOLENATE |
Origen del producto |
United States |
Retrosynthesis Analysis
AI-Powered Synthesis Planning: Our tool employs the Template_relevance Pistachio, Template_relevance Bkms_metabolic, Template_relevance Pistachio_ringbreaker, Template_relevance Reaxys, Template_relevance Reaxys_biocatalysis model, leveraging a vast database of chemical reactions to predict feasible synthetic routes.
One-Step Synthesis Focus: Specifically designed for one-step synthesis, it provides concise and direct routes for your target compounds, streamlining the synthesis process.
Accurate Predictions: Utilizing the extensive PISTACHIO, BKMS_METABOLIC, PISTACHIO_RINGBREAKER, REAXYS, REAXYS_BIOCATALYSIS database, our tool offers high-accuracy predictions, reflecting the latest in chemical research and data.
Strategy Settings
Precursor scoring | Relevance Heuristic |
---|---|
Min. plausibility | 0.01 |
Model | Template_relevance |
Template Set | Pistachio/Bkms_metabolic/Pistachio_ringbreaker/Reaxys/Reaxys_biocatalysis |
Top-N result to add to graph | 6 |
Feasible Synthetic Routes
Descargo de responsabilidad e información sobre productos de investigación in vitro
Tenga en cuenta que todos los artículos e información de productos presentados en BenchChem están destinados únicamente con fines informativos. Los productos disponibles para la compra en BenchChem están diseñados específicamente para estudios in vitro, que se realizan fuera de organismos vivos. Los estudios in vitro, derivados del término latino "in vidrio", involucran experimentos realizados en entornos de laboratorio controlados utilizando células o tejidos. Es importante tener en cuenta que estos productos no se clasifican como medicamentos y no han recibido la aprobación de la FDA para la prevención, tratamiento o cura de ninguna condición médica, dolencia o enfermedad. Debemos enfatizar que cualquier forma de introducción corporal de estos productos en humanos o animales está estrictamente prohibida por ley. Es esencial adherirse a estas pautas para garantizar el cumplimiento de los estándares legales y éticos en la investigación y experimentación.