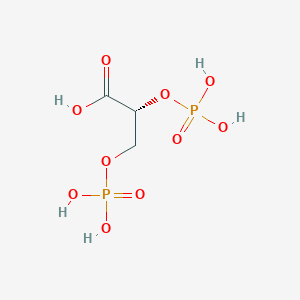
2,3-bisphospho-D-glycerate
Descripción general
Descripción
2,3-Bisphospho-D-glycerate is a three-carbon isomer of the glycolytic intermediate 1,3-bisphosphoglyceric acid. It is present in human red blood cells at approximately 5 mmol/L. This compound plays a crucial role in regulating oxygen release from hemoglobin by binding with greater affinity to deoxygenated hemoglobin than to oxygenated hemoglobin .
Aplicaciones Científicas De Investigación
2,3-Bisphospho-D-glycerate has several important applications in scientific research:
Mecanismo De Acción
2,3-BPG binds with greater affinity to deoxygenated hemoglobin than it does to oxygenated hemoglobin . It interacts with deoxygenated hemoglobin beta subunits and decreases the affinity for oxygen and allosterically promotes the release of the remaining oxygen molecules bound to the hemoglobin . Therefore, it enhances the ability of red blood cells to release oxygen near tissues that need it most .
Métodos De Preparación
Synthetic Routes and Reaction Conditions
2,3-Bisphospho-D-glycerate is synthesized from 1,3-bisphosphoglyceric acid by the enzyme bisphosphoglycerate mutase. This enzyme catalyzes the transfer of a phosphoryl group from the C1 position to the C2 position of 1,3-bisphosphoglyceric acid, resulting in the formation of this compound .
Industrial Production Methods
Industrial production of this compound is not common due to its specific biological role and the complexity of its synthesis. It is typically produced in laboratory settings for research purposes.
Análisis De Reacciones Químicas
Types of Reactions
2,3-Bisphospho-D-glycerate primarily undergoes hydrolysis and phosphorylation reactions. It can be hydrolyzed by bisphosphoglycerate phosphatase to form 3-phosphoglycerate and inorganic phosphate .
Common Reagents and Conditions
Hydrolysis: Catalyzed by bisphosphoglycerate phosphatase in the presence of water.
Phosphorylation: Involves the transfer of a phosphoryl group by bisphosphoglycerate mutase.
Major Products
Hydrolysis: 3-phosphoglycerate and inorganic phosphate.
Phosphorylation: Formation of this compound from 1,3-bisphosphoglyceric acid.
Comparación Con Compuestos Similares
Similar Compounds
1,3-Bisphosphoglyceric acid: A glycolytic intermediate that can be converted into 2,3-bisphospho-D-glycerate by bisphosphoglycerate mutase.
3-Phosphoglycerate: A product of the hydrolysis of this compound.
Uniqueness
This compound is unique in its ability to regulate oxygen release from hemoglobin, a function not shared by its isomers. Its role as an allosteric effector makes it essential for maintaining efficient oxygen delivery under varying physiological conditions .
Propiedades
IUPAC Name |
(2R)-2,3-diphosphonooxypropanoic acid | |
---|---|---|
Source | PubChem | |
URL | https://pubchem.ncbi.nlm.nih.gov | |
Description | Data deposited in or computed by PubChem | |
InChI |
InChI=1S/C3H8O10P2/c4-3(5)2(13-15(9,10)11)1-12-14(6,7)8/h2H,1H2,(H,4,5)(H2,6,7,8)(H2,9,10,11)/t2-/m1/s1 | |
Source | PubChem | |
URL | https://pubchem.ncbi.nlm.nih.gov | |
Description | Data deposited in or computed by PubChem | |
InChI Key |
XOHUEYCVLUUEJJ-UWTATZPHSA-N | |
Source | PubChem | |
URL | https://pubchem.ncbi.nlm.nih.gov | |
Description | Data deposited in or computed by PubChem | |
Canonical SMILES |
C(C(C(=O)O)OP(=O)(O)O)OP(=O)(O)O | |
Source | PubChem | |
URL | https://pubchem.ncbi.nlm.nih.gov | |
Description | Data deposited in or computed by PubChem | |
Isomeric SMILES |
C([C@H](C(=O)O)OP(=O)(O)O)OP(=O)(O)O | |
Source | PubChem | |
URL | https://pubchem.ncbi.nlm.nih.gov | |
Description | Data deposited in or computed by PubChem | |
Molecular Formula |
C3H8O10P2 | |
Source | PubChem | |
URL | https://pubchem.ncbi.nlm.nih.gov | |
Description | Data deposited in or computed by PubChem | |
Molecular Weight |
266.04 g/mol | |
Source | PubChem | |
URL | https://pubchem.ncbi.nlm.nih.gov | |
Description | Data deposited in or computed by PubChem | |
Physical Description |
Solid | |
Record name | 2,3-Diphosphoglyceric acid | |
Source | Human Metabolome Database (HMDB) | |
URL | http://www.hmdb.ca/metabolites/HMDB0001294 | |
Description | The Human Metabolome Database (HMDB) is a freely available electronic database containing detailed information about small molecule metabolites found in the human body. | |
Explanation | HMDB is offered to the public as a freely available resource. Use and re-distribution of the data, in whole or in part, for commercial purposes requires explicit permission of the authors and explicit acknowledgment of the source material (HMDB) and the original publication (see the HMDB citing page). We ask that users who download significant portions of the database cite the HMDB paper in any resulting publications. | |
CAS No. |
14438-19-8 | |
Record name | Glyceric acid, bis(dihydrogen phosphate), D- | |
Source | ChemIDplus | |
URL | https://pubchem.ncbi.nlm.nih.gov/substance/?source=chemidplus&sourceid=0014438198 | |
Description | ChemIDplus is a free, web search system that provides access to the structure and nomenclature authority files used for the identification of chemical substances cited in National Library of Medicine (NLM) databases, including the TOXNET system. | |
Record name | 2,3-Diphosphoglyceric acid | |
Source | Human Metabolome Database (HMDB) | |
URL | http://www.hmdb.ca/metabolites/HMDB0001294 | |
Description | The Human Metabolome Database (HMDB) is a freely available electronic database containing detailed information about small molecule metabolites found in the human body. | |
Explanation | HMDB is offered to the public as a freely available resource. Use and re-distribution of the data, in whole or in part, for commercial purposes requires explicit permission of the authors and explicit acknowledgment of the source material (HMDB) and the original publication (see the HMDB citing page). We ask that users who download significant portions of the database cite the HMDB paper in any resulting publications. | |
Retrosynthesis Analysis
AI-Powered Synthesis Planning: Our tool employs the Template_relevance Pistachio, Template_relevance Bkms_metabolic, Template_relevance Pistachio_ringbreaker, Template_relevance Reaxys, Template_relevance Reaxys_biocatalysis model, leveraging a vast database of chemical reactions to predict feasible synthetic routes.
One-Step Synthesis Focus: Specifically designed for one-step synthesis, it provides concise and direct routes for your target compounds, streamlining the synthesis process.
Accurate Predictions: Utilizing the extensive PISTACHIO, BKMS_METABOLIC, PISTACHIO_RINGBREAKER, REAXYS, REAXYS_BIOCATALYSIS database, our tool offers high-accuracy predictions, reflecting the latest in chemical research and data.
Strategy Settings
Precursor scoring | Relevance Heuristic |
---|---|
Min. plausibility | 0.01 |
Model | Template_relevance |
Template Set | Pistachio/Bkms_metabolic/Pistachio_ringbreaker/Reaxys/Reaxys_biocatalysis |
Top-N result to add to graph | 6 |
Feasible Synthetic Routes
Q1: How does 2,3-bisphospho-D-glycerate interact with phosphoglycerate mutase, and what are the downstream effects?
A: this compound acts as a cofactor for certain phosphoglycerate mutase enzymes, but not for the enzyme derived from wheat germ. Research using pig-kidney phosphoglycerate mutase demonstrated that this enzyme utilizes a "phosphoenzyme" mechanism []. This involves the transfer of a phosphate group from this compound to the enzyme, forming a phosphorylated enzyme intermediate. This phosphate group is then transferred to the substrate, 2-phospho-D-glycerate, converting it to 3-phospho-D-glycerate. This reaction is crucial in glycolysis, ultimately impacting energy production within the cell.
Q2: The research mentions the use of 18O-labeled substrates. What is the significance of this technique in studying phosphoglycerate mutase?
A: Using 18O-labeled phospho-D-glycerate and H2(18)O allowed researchers to track the fate of oxygen atoms during the reaction catalyzed by wheat germ phosphoglycerate mutase []. The findings definitively showed that the isomerization of 2-phospho-D-glycerate and 3-phospho-D-glycerate does not proceed through a 2,3-cyclic phosphate intermediate. This elegant isotopic labeling experiment provided crucial evidence for a mechanism involving a direct phosphoryl transfer via a phosphoryl-enzyme intermediate.
Q3: Besides its role in glycolysis, does this compound interact with other proteins?
A: Yes, research indicates that this compound interacts with dromedary hemoglobin []. In this case, it acts as an allosteric effector, binding to the hemoglobin molecule and influencing its oxygen affinity. This interaction, alongside chloride and other solvent components, highlights the intricate interplay between this compound and protein structure and function beyond its well-known role in glycolysis.
Descargo de responsabilidad e información sobre productos de investigación in vitro
Tenga en cuenta que todos los artículos e información de productos presentados en BenchChem están destinados únicamente con fines informativos. Los productos disponibles para la compra en BenchChem están diseñados específicamente para estudios in vitro, que se realizan fuera de organismos vivos. Los estudios in vitro, derivados del término latino "in vidrio", involucran experimentos realizados en entornos de laboratorio controlados utilizando células o tejidos. Es importante tener en cuenta que estos productos no se clasifican como medicamentos y no han recibido la aprobación de la FDA para la prevención, tratamiento o cura de ninguna condición médica, dolencia o enfermedad. Debemos enfatizar que cualquier forma de introducción corporal de estos productos en humanos o animales está estrictamente prohibida por ley. Es esencial adherirse a estas pautas para garantizar el cumplimiento de los estándares legales y éticos en la investigación y experimentación.