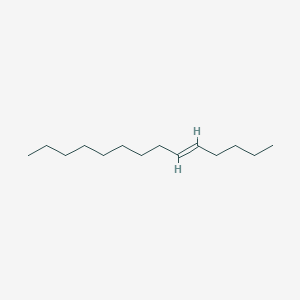
(E)-5-Tetradecene
Descripción general
Descripción
(E)-5-Tetradecene: is an organic compound with the molecular formula C14H28 . It is a type of alkene, specifically a long-chain hydrocarbon with a double bond located at the fifth carbon atom in the chain. The “(E)” designation indicates that the compound has a trans configuration around the double bond, meaning the hydrogen atoms are on opposite sides of the double bond. This compound is also known by other names such as This compound and trans-5-Tetradecene .
Métodos De Preparación
Synthetic Routes and Reaction Conditions: (E)-5-Tetradecene can be synthesized through various methods, including:
Dehydration of Alcohols: One common method involves the dehydration of tetradecanol using an acid catalyst such as sulfuric acid. The reaction typically occurs under elevated temperatures to facilitate the removal of water and formation of the double bond.
Wittig Reaction: Another method involves the Wittig reaction, where a phosphonium ylide reacts with an aldehyde or ketone to form the desired alkene. This method allows for precise control over the position and configuration of the double bond.
Industrial Production Methods: In industrial settings, this compound is often produced through the cracking of petroleum hydrocarbons . This process involves breaking down larger hydrocarbon molecules into smaller ones, including alkenes like this compound. The reaction conditions typically involve high temperatures and pressures, along with the use of catalysts to enhance the efficiency of the process .
Análisis De Reacciones Químicas
Types of Reactions:
Oxidation: (E)-5-Tetradecene can undergo oxidation reactions to form various oxygenated compounds such as alcohols, aldehydes, and carboxylic acids. Common oxidizing agents include potassium permanganate and chromium trioxide.
Reduction: The compound can be reduced to form the corresponding alkane, tetradecane, using reducing agents like hydrogen gas in the presence of a metal catalyst such as palladium or platinum.
Addition Reactions: this compound readily undergoes addition reactions due to the presence of the double bond. For example, it can react with hydrogen halides (e.g., hydrogen chloride) to form haloalkanes or with halogens (e.g., bromine) to form dihaloalkanes.
Polymerization: Under certain conditions, this compound can undergo polymerization to form long-chain polymers. This reaction is typically catalyzed by metal catalysts such as Ziegler-Natta catalysts.
Common Reagents and Conditions:
Oxidation: Potassium permanganate (KMnO4), chromium trioxide (CrO3)
Reduction: Hydrogen gas (H2) with palladium (Pd) or platinum (Pt) catalysts
Addition: Hydrogen halides (HCl, HBr), halogens (Br2, Cl2)
Polymerization: Ziegler-Natta catalysts
Major Products:
Oxidation: Alcohols, aldehydes, carboxylic acids
Reduction: Tetradecane
Addition: Haloalkanes, dihaloalkanes
Polymerization: Long-chain polymers
Aplicaciones Científicas De Investigación
Chemistry: (E)-5-Tetradecene is used as a starting material in organic synthesis to produce various other compounds. Its reactivity due to the double bond makes it a valuable intermediate in the synthesis of complex molecules.
Biology and Medicine: In biological research, this compound is used to study the effects of alkenes on biological systems . It can serve as a model compound to understand the behavior of similar alkenes in biological membranes and their interactions with proteins and other biomolecules.
Industry: In the industrial sector, this compound is used as a monomer in the production of polymers and copolymers. These materials have applications in the manufacture of plastics, adhesives, and coatings. Additionally, it is used as a solvent and in the formulation of lubricants and surfactants .
Mecanismo De Acción
The mechanism of action of (E)-5-Tetradecene primarily involves its reactivity at the double bond . The double bond can participate in various chemical reactions, such as addition and polymerization, which are facilitated by the presence of catalysts or specific reaction conditions. In biological systems, the compound can interact with lipid membranes and proteins , affecting their structure and function. The exact molecular targets and pathways involved depend on the specific context and application of the compound .
Comparación Con Compuestos Similares
5-Tetradecene, (Z)-: This is the cis isomer of 5-Tetradecene, where the hydrogen atoms are on the same side of the double bond. The different spatial arrangement can lead to variations in physical and chemical properties.
1-Tetradecene: This compound has the double bond at the first carbon atom in the chain, resulting in different reactivity and applications.
Tetradecane: This is the fully saturated alkane with no double bonds, leading to different chemical behavior and uses.
Uniqueness of (E)-5-Tetradecene: The trans configuration of this compound imparts unique physical properties, such as a higher melting point compared to its cis isomer. Its specific reactivity at the fifth carbon position makes it a valuable intermediate in organic synthesis and industrial applications .
Actividad Biológica
(E)-5-Tetradecene, a straight-chain alkene with the chemical formula CH, has garnered attention for its diverse biological activities. This article explores its antimicrobial properties, ecological roles, and potential applications in various fields, supported by recent research findings.
Chemical Structure and Properties
This compound is characterized by a double bond at the fifth carbon position of a tetradecane chain. Its structural formula can be represented as follows:
The presence of the double bond contributes to its reactivity and biological interactions.
Antimicrobial Activity
Recent studies have highlighted the antimicrobial potential of tetradecane derivatives, including this compound. A notable study extracted tetradecane from Pediococcus acidilactici, demonstrating significant antibacterial and antifungal activities against various pathogens such as Staphylococcus aureus and Candida albicans. The minimum inhibitory concentrations (MICs) were found to be notably low, indicating strong bioactivity .
Table 1: Antimicrobial Efficacy of this compound
Pathogen | MIC (mg/mL) | Activity Type |
---|---|---|
Staphylococcus aureus | 0.5 | Antibacterial |
Escherichia coli | 0.8 | Antibacterial |
Candida albicans | 1.0 | Antifungal |
This study is pivotal as it marks one of the first reports of tetradecane's antimicrobial properties derived from lactic acid bacteria, suggesting its potential as a natural preservative or therapeutic agent.
Ecological Roles
In addition to its antimicrobial properties, this compound plays a significant role in chemical ecology . Research has shown that this compound acts as an attractant for certain insect species, particularly mirid bugs. In controlled experiments, female A. lucorum exhibited a strong preference for host plants emitting this compound over others, highlighting its importance in plant-insect interactions .
Table 2: Attractiveness of this compound to Insects
Compound | Number of Bugs Trapped | Concentration (mg/mL) |
---|---|---|
This compound | 30.33 ± 2.19 | 1.5 |
Control Group | 10.00 ± 1.50 | N/A |
This ecological function suggests that this compound could be utilized in pest management strategies by enhancing the attractiveness of traps or baits.
Case Studies and Research Findings
- Antimicrobial Potential : A study on the extraction and characterization of tetradecane from Pediococcus acidilactici confirmed its bioactive properties through various assays including disc diffusion and MIC determination .
- Insect Behavior : Another investigation into the olfactory responses of mirid bugs indicated that this compound was significantly more attractive than other tested compounds, emphasizing its ecological significance in attracting beneficial insects .
- Pharmacological Applications : While direct pharmacological applications of this compound remain underexplored, its antimicrobial properties suggest potential use in developing new antibiotics or preservatives, especially in food technology .
Propiedades
IUPAC Name |
(E)-tetradec-5-ene | |
---|---|---|
Source | PubChem | |
URL | https://pubchem.ncbi.nlm.nih.gov | |
Description | Data deposited in or computed by PubChem | |
InChI |
InChI=1S/C14H28/c1-3-5-7-9-11-13-14-12-10-8-6-4-2/h9,11H,3-8,10,12-14H2,1-2H3/b11-9+ | |
Source | PubChem | |
URL | https://pubchem.ncbi.nlm.nih.gov | |
Description | Data deposited in or computed by PubChem | |
InChI Key |
SNIFAVVHRQZYGO-PKNBQFBNSA-N | |
Source | PubChem | |
URL | https://pubchem.ncbi.nlm.nih.gov | |
Description | Data deposited in or computed by PubChem | |
Canonical SMILES |
CCCCCCCCC=CCCCC | |
Source | PubChem | |
URL | https://pubchem.ncbi.nlm.nih.gov | |
Description | Data deposited in or computed by PubChem | |
Isomeric SMILES |
CCCCCCCC/C=C/CCCC | |
Source | PubChem | |
URL | https://pubchem.ncbi.nlm.nih.gov | |
Description | Data deposited in or computed by PubChem | |
Molecular Formula |
C14H28 | |
Source | PubChem | |
URL | https://pubchem.ncbi.nlm.nih.gov | |
Description | Data deposited in or computed by PubChem | |
DSSTOX Substance ID |
DTXSID50880854 | |
Record name | 5-tetradecene, (e)- | |
Source | EPA DSSTox | |
URL | https://comptox.epa.gov/dashboard/DTXSID50880854 | |
Description | DSSTox provides a high quality public chemistry resource for supporting improved predictive toxicology. | |
Molecular Weight |
196.37 g/mol | |
Source | PubChem | |
URL | https://pubchem.ncbi.nlm.nih.gov | |
Description | Data deposited in or computed by PubChem | |
CAS No. |
41446-66-6 | |
Record name | 5-Tetradecene, (5E)- | |
Source | ChemIDplus | |
URL | https://pubchem.ncbi.nlm.nih.gov/substance/?source=chemidplus&sourceid=0041446666 | |
Description | ChemIDplus is a free, web search system that provides access to the structure and nomenclature authority files used for the identification of chemical substances cited in National Library of Medicine (NLM) databases, including the TOXNET system. | |
Record name | 5-tetradecene, (e)- | |
Source | EPA DSSTox | |
URL | https://comptox.epa.gov/dashboard/DTXSID50880854 | |
Description | DSSTox provides a high quality public chemistry resource for supporting improved predictive toxicology. | |
Record name | 5-TETRADECENE, (5E)- | |
Source | FDA Global Substance Registration System (GSRS) | |
URL | https://gsrs.ncats.nih.gov/ginas/app/beta/substances/6MV8XMM426 | |
Description | The FDA Global Substance Registration System (GSRS) enables the efficient and accurate exchange of information on what substances are in regulated products. Instead of relying on names, which vary across regulatory domains, countries, and regions, the GSRS knowledge base makes it possible for substances to be defined by standardized, scientific descriptions. | |
Explanation | Unless otherwise noted, the contents of the FDA website (www.fda.gov), both text and graphics, are not copyrighted. They are in the public domain and may be republished, reprinted and otherwise used freely by anyone without the need to obtain permission from FDA. Credit to the U.S. Food and Drug Administration as the source is appreciated but not required. | |
Retrosynthesis Analysis
AI-Powered Synthesis Planning: Our tool employs the Template_relevance Pistachio, Template_relevance Bkms_metabolic, Template_relevance Pistachio_ringbreaker, Template_relevance Reaxys, Template_relevance Reaxys_biocatalysis model, leveraging a vast database of chemical reactions to predict feasible synthetic routes.
One-Step Synthesis Focus: Specifically designed for one-step synthesis, it provides concise and direct routes for your target compounds, streamlining the synthesis process.
Accurate Predictions: Utilizing the extensive PISTACHIO, BKMS_METABOLIC, PISTACHIO_RINGBREAKER, REAXYS, REAXYS_BIOCATALYSIS database, our tool offers high-accuracy predictions, reflecting the latest in chemical research and data.
Strategy Settings
Precursor scoring | Relevance Heuristic |
---|---|
Min. plausibility | 0.01 |
Model | Template_relevance |
Template Set | Pistachio/Bkms_metabolic/Pistachio_ringbreaker/Reaxys/Reaxys_biocatalysis |
Top-N result to add to graph | 6 |
Feasible Synthetic Routes
Descargo de responsabilidad e información sobre productos de investigación in vitro
Tenga en cuenta que todos los artículos e información de productos presentados en BenchChem están destinados únicamente con fines informativos. Los productos disponibles para la compra en BenchChem están diseñados específicamente para estudios in vitro, que se realizan fuera de organismos vivos. Los estudios in vitro, derivados del término latino "in vidrio", involucran experimentos realizados en entornos de laboratorio controlados utilizando células o tejidos. Es importante tener en cuenta que estos productos no se clasifican como medicamentos y no han recibido la aprobación de la FDA para la prevención, tratamiento o cura de ninguna condición médica, dolencia o enfermedad. Debemos enfatizar que cualquier forma de introducción corporal de estos productos en humanos o animales está estrictamente prohibida por ley. Es esencial adherirse a estas pautas para garantizar el cumplimiento de los estándares legales y éticos en la investigación y experimentación.