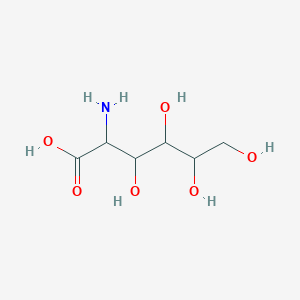
Ácido D-Glucosamínico
Descripción general
Descripción
El ácido glucosamínico, también conocido como ácido 2-amino-2-desoxi-D-glucónico, es un derivado de la glucosamina. Es un compuesto valioso en el campo de la bioquímica debido a su papel en varios procesos bioquímicos. El ácido glucosamínico es un componente de los lipopolisacáridos bacterianos y participa en la biosíntesis de proteínas y lípidos glicosilados .
Aplicaciones Científicas De Investigación
El ácido glucosamínico tiene una amplia gama de aplicaciones en la investigación científica:
Mecanismo De Acción
El mecanismo de acción del ácido glucosamínico implica su papel como precursor en la biosíntesis de proteínas y lípidos glicosilados. Participa en diversas vías bioquímicas, incluida la síntesis de lipopolisacáridos bacterianos. La enzima glucosamina oxidasa cataliza la oxidación de la glucosamina a ácido glucosamínico, que luego participa en reacciones bioquímicas adicionales .
Compuestos Similares:
Glucosamina: Un precursor del ácido glucosamínico, involucrado en la biosíntesis de proteínas y lípidos glicosilados.
N-acetilglucosamina: Otro derivado de la glucosamina, utilizado en la síntesis de glucosaminoglucanos y proteoglicanos.
Quitosamina: Un componente de la quitina y el quitosano, estructuralmente similar a la glucosamina.
Unicidad: El ácido glucosamínico es único debido a su papel específico en la oxidación de la glucosamina y sus aplicaciones en la síntesis de compuestos quirales e inhibidores de glucosidasas. Su alta especificidad de sustrato y estabilidad en diversas condiciones lo convierten en un compuesto valioso en la investigación bioquímica y las aplicaciones industriales .
Análisis Bioquímico
Biochemical Properties
It is known that D-Glucosaminic acid can be utilized by certain bacteria such as Salmonella enterica . The exact enzymes, proteins, and other biomolecules it interacts with are yet to be identified.
Cellular Effects
It has been suggested that D-Glucosaminic acid may contribute to cell membrane stability and regenerative medicine . It has been shown to have superoxide/hydroxyl radical scavenging activities, a strong chelating effect on ferrous ions, and enhances the reduced glutathione level to promote activity against intracellular oxidative stress .
Molecular Mechanism
It is known that D-Glucosaminic acid is transported across the cell membrane and phosphorylated at the C-6 position during this process
Temporal Effects in Laboratory Settings
It has been suggested that D-Glucosaminic acid may have a role in wound healing, promoting cell proliferation and differentiation .
Metabolic Pathways
It is known that D-Glucosaminic acid is involved in the metabolism of certain bacteria , but the specific enzymes or cofactors it interacts with, and its effects on metabolic flux or metabolite levels, are yet to be identified.
Transport and Distribution
D-Glucosaminic acid is transported across the cell membrane by a phosphotransferase system (PTS) permease
Subcellular Localization
It is known that D-Glucosaminic acid is transported across the cell membrane , but whether it has any targeting signals or post-translational modifications that direct it to specific compartments or organelles is yet to be identified.
Métodos De Preparación
Rutas Sintéticas y Condiciones de Reacción: El ácido glucosamínico se puede sintetizar mediante la oxidación de la glucosamina. Un método eficiente implica el uso de catalizadores de oro soportados para la oxidación con aire sin base de la glucosamina. Este proceso se lleva a cabo en agua a temperatura cercana a la ambiente y aire atmosférico, logrando un alto rendimiento de ácido glucosamínico .
Métodos de Producción Industrial: La producción industrial de ácido glucosamínico a menudo implica la fermentación de ciertos microorganismos. Por ejemplo, la cepa fúngica Aspergillus terreus NUK-1204 se ha utilizado para producir ácido glucosamínico mediante la oxidación de la glucosamina. La enzima glucosamina oxidasa, derivada de esta cepa, exhibe alta especificidad de sustrato y estabilidad en diversas condiciones de pH y temperatura .
Análisis De Reacciones Químicas
Tipos de Reacciones: El ácido glucosamínico experimenta varios tipos de reacciones químicas, incluidas la oxidación, la reducción y la sustitución. La oxidación de la glucosamina a ácido glucosamínico es una reacción notable, a menudo catalizada por enzimas o catalizadores metálicos soportados .
Reactivos y Condiciones Comunes:
Oxidación: Catalizadores de oro soportados en agua a temperatura cercana a la ambiente y aire atmosférico.
Reducción: Se pueden utilizar agentes reductores específicos para convertir el ácido glucosamínico de nuevo a glucosamina.
Sustitución: Diversos nucleófilos pueden reaccionar con el ácido glucosamínico para formar derivados sustituidos.
Productos Principales: El producto principal de la oxidación de la glucosamina es el ácido glucosamínico. Otras reacciones pueden producir varios derivados sustituidos dependiendo de los reactivos y las condiciones utilizados.
Comparación Con Compuestos Similares
Glucosamine: A precursor to glucosaminic acid, involved in the biosynthesis of glycosylated proteins and lipids.
N-acetylglucosamine: Another derivative of glucosamine, used in the synthesis of glycosaminoglycans and proteoglycans.
Chitosamine: A component of chitosan and chitin, structurally similar to glucosamine.
Uniqueness: Glucosaminic acid is unique due to its specific role in the oxidation of glucosamine and its applications in the synthesis of chiral compounds and glycosidase inhibitors. Its high substrate specificity and stability under various conditions make it a valuable compound in biochemical research and industrial applications .
Actividad Biológica
D-Glucosaminic acid (DGA) is an amino sugar that plays a significant role in various biological processes. Its structural similarity to D-glucosamine enables it to participate in metabolic pathways and exhibit diverse biological activities, making it a subject of interest in pharmaceutical and biomedical research.
Chemical Structure and Properties
D-Glucosaminic acid is a derivative of D-glucosamine, characterized by the presence of a carboxylic acid group at the C-2 position. This modification alters its chemical properties, enhancing its potential biological activities. The molecular formula for D-glucosaminic acid is C₆H₁₃NO₅, and it has a molar mass of approximately 175.18 g/mol.
1. Metabolic Role
Recent studies have highlighted the metabolic pathway of D-glucosaminic acid in Salmonella enterica serovar Typhimurium, where it undergoes phosphorylation at C-6 to form D-glucosaminate-6-phosphate (DGA-6-P). This compound is crucial as it participates in the Entner-Doudoroff pathway, which is essential for bacterial metabolism and pathogenicity .
2. Antidiabetic Effects
D-glucosaminic acid has been investigated for its antidiabetic properties. A study on chromium(III) complexes of D-glucosaminic acid demonstrated significant blood sugar-lowering effects in type-2 diabetes model rats. The results indicated that these complexes could effectively reduce blood glucose levels, comparable to known antidiabetic agents .
Study | Compound | Model | Effect |
---|---|---|---|
Chromium(III) Complexes | D-Glucosaminic Acid | Type-2 Diabetes Rats | Decreased blood sugar levels |
3. Hypocholesterolemic Effects
The hypocholesterolemic properties of D-glucosaminic acid have been explored through its derivatives, particularly chitosan. Research indicates that low molecular weight chitosans derived from D-glucosaminic acid can lower plasma lipid levels and improve cholesterol profiles in animal models. The mechanism involves enhanced binding capacities to fats and bile acids, promoting their excretion .
Case Study 1: Chitosan Derivatives
A study focused on enzymatically prepared low molecular weight chitosans derived from D-glucosaminic acid showed significant hypocholesterolemic effects in rats. The results highlighted that variations in degree of acetylation (DA) and molecular weight (Mw) significantly influenced lipid-binding capacities and overall cholesterol reduction .
Case Study 2: Metabolic Pathways
Research into the enzymatic pathways involving D-glucosaminic acid revealed its conversion into bioactive metabolites that are essential for bacterial survival and virulence. The identification of enzymes such as D-glucosaminate-6-phosphate ammonia-lyase (DGL) underscores its importance in microbial metabolism .
Future Directions
The potential applications of D-glucosaminic acid extend into pharmaceuticals, particularly for developing new treatments for diabetes and hyperlipidemia. Further research is warranted to explore its efficacy in human clinical trials and to understand the underlying mechanisms governing its biological activities.
Propiedades
IUPAC Name |
2-amino-3,4,5,6-tetrahydroxyhexanoic acid | |
---|---|---|
Details | Computed by LexiChem 2.6.6 (PubChem release 2019.06.18) | |
Source | PubChem | |
URL | https://pubchem.ncbi.nlm.nih.gov | |
Description | Data deposited in or computed by PubChem | |
InChI |
InChI=1S/C6H13NO6/c7-3(6(12)13)5(11)4(10)2(9)1-8/h2-5,8-11H,1,7H2,(H,12,13) | |
Details | Computed by InChI 1.0.5 (PubChem release 2019.06.18) | |
Source | PubChem | |
URL | https://pubchem.ncbi.nlm.nih.gov | |
Description | Data deposited in or computed by PubChem | |
InChI Key |
UFYKDFXCZBTLOO-UHFFFAOYSA-N | |
Details | Computed by InChI 1.0.5 (PubChem release 2019.06.18) | |
Source | PubChem | |
URL | https://pubchem.ncbi.nlm.nih.gov | |
Description | Data deposited in or computed by PubChem | |
Canonical SMILES |
C(C(C(C(C(C(=O)O)N)O)O)O)O | |
Details | Computed by OEChem 2.1.5 (PubChem release 2019.06.18) | |
Source | PubChem | |
URL | https://pubchem.ncbi.nlm.nih.gov | |
Description | Data deposited in or computed by PubChem | |
Molecular Formula |
C6H13NO6 | |
Details | Computed by PubChem 2.1 (PubChem release 2019.06.18) | |
Source | PubChem | |
URL | https://pubchem.ncbi.nlm.nih.gov | |
Description | Data deposited in or computed by PubChem | |
Molecular Weight |
195.17 g/mol | |
Details | Computed by PubChem 2.1 (PubChem release 2021.05.07) | |
Source | PubChem | |
URL | https://pubchem.ncbi.nlm.nih.gov | |
Description | Data deposited in or computed by PubChem | |
CAS No. |
3646-68-2 | |
Record name | Glucosaminic acid | |
Source | DTP/NCI | |
URL | https://dtp.cancer.gov/dtpstandard/servlet/dwindex?searchtype=NSC&outputformat=html&searchlist=404265 | |
Description | The NCI Development Therapeutics Program (DTP) provides services and resources to the academic and private-sector research communities worldwide to facilitate the discovery and development of new cancer therapeutic agents. | |
Explanation | Unless otherwise indicated, all text within NCI products is free of copyright and may be reused without our permission. Credit the National Cancer Institute as the source. | |
Record name | Glucosaminic acid | |
Source | DTP/NCI | |
URL | https://dtp.cancer.gov/dtpstandard/servlet/dwindex?searchtype=NSC&outputformat=html&searchlist=37779 | |
Description | The NCI Development Therapeutics Program (DTP) provides services and resources to the academic and private-sector research communities worldwide to facilitate the discovery and development of new cancer therapeutic agents. | |
Explanation | Unless otherwise indicated, all text within NCI products is free of copyright and may be reused without our permission. Credit the National Cancer Institute as the source. | |
Retrosynthesis Analysis
AI-Powered Synthesis Planning: Our tool employs the Template_relevance Pistachio, Template_relevance Bkms_metabolic, Template_relevance Pistachio_ringbreaker, Template_relevance Reaxys, Template_relevance Reaxys_biocatalysis model, leveraging a vast database of chemical reactions to predict feasible synthetic routes.
One-Step Synthesis Focus: Specifically designed for one-step synthesis, it provides concise and direct routes for your target compounds, streamlining the synthesis process.
Accurate Predictions: Utilizing the extensive PISTACHIO, BKMS_METABOLIC, PISTACHIO_RINGBREAKER, REAXYS, REAXYS_BIOCATALYSIS database, our tool offers high-accuracy predictions, reflecting the latest in chemical research and data.
Strategy Settings
Precursor scoring | Relevance Heuristic |
---|---|
Min. plausibility | 0.01 |
Model | Template_relevance |
Template Set | Pistachio/Bkms_metabolic/Pistachio_ringbreaker/Reaxys/Reaxys_biocatalysis |
Top-N result to add to graph | 6 |
Feasible Synthetic Routes
Descargo de responsabilidad e información sobre productos de investigación in vitro
Tenga en cuenta que todos los artículos e información de productos presentados en BenchChem están destinados únicamente con fines informativos. Los productos disponibles para la compra en BenchChem están diseñados específicamente para estudios in vitro, que se realizan fuera de organismos vivos. Los estudios in vitro, derivados del término latino "in vidrio", involucran experimentos realizados en entornos de laboratorio controlados utilizando células o tejidos. Es importante tener en cuenta que estos productos no se clasifican como medicamentos y no han recibido la aprobación de la FDA para la prevención, tratamiento o cura de ninguna condición médica, dolencia o enfermedad. Debemos enfatizar que cualquier forma de introducción corporal de estos productos en humanos o animales está estrictamente prohibida por ley. Es esencial adherirse a estas pautas para garantizar el cumplimiento de los estándares legales y éticos en la investigación y experimentación.