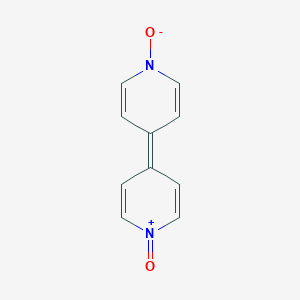
4,4'-Bipyridine 1,1'-dioxide
Descripción general
Descripción
4,4’-Bipyridine 1,1’-dioxide is an organic compound with the molecular formula C10H8N2O2. It is a derivative of bipyridine, where both nitrogen atoms are oxidized to form N-oxides. This compound is known for its unique chemical properties and has found applications in various fields, including coordination chemistry, catalysis, and materials science.
Métodos De Preparación
Synthetic Routes and Reaction Conditions
4,4’-Bipyridine 1,1’-dioxide can be synthesized through several methods. One common approach involves the oxidation of 4,4’-bipyridine using hydrogen peroxide or peracids. The reaction typically proceeds under mild conditions, with the bipyridine dissolved in an appropriate solvent such as acetic acid or methanol. The reaction is usually carried out at room temperature or slightly elevated temperatures to ensure complete oxidation.
Another method involves the use of 4,4’-bipyridine N,N’-dichloride as a starting material, which is then treated with hydrogen peroxide to yield 4,4’-bipyridine 1,1’-dioxide .
Industrial Production Methods
In an industrial setting, the production of 4,4’-bipyridine 1,1’-dioxide may involve continuous flow processes to ensure high yield and purity. The use of catalysts and optimized reaction conditions can enhance the efficiency of the oxidation process. Additionally, purification steps such as recrystallization or chromatography may be employed to obtain the final product with the desired purity.
Análisis De Reacciones Químicas
Types of Reactions
4,4’-Bipyridine 1,1’-dioxide undergoes various chemical reactions, including:
Oxidation: Further oxidation can lead to the formation of higher oxidation state compounds.
Reduction: The compound can be reduced back to 4,4’-bipyridine under appropriate conditions.
Common Reagents and Conditions
Oxidation: Hydrogen peroxide, peracids.
Reduction: Metal hydrides, catalytic hydrogenation.
Substitution: Halogenating agents, nitrating agents.
Major Products Formed
Oxidation: Higher oxidation state derivatives.
Reduction: 4,4’-Bipyridine.
Substitution: Functionalized bipyridine derivatives with various substituents.
Aplicaciones Científicas De Investigación
Coordination Chemistry
One of the most significant applications of 4,4'-bipyridine 1,1'-dioxide is as a ligand in coordination chemistry. It forms stable complexes with various metal ions, which are crucial for synthesizing metal-organic frameworks (MOFs) and catalysts. The nitrogen atoms in the bipyridine rings serve as electron donors, allowing for the formation of unique electronic and magnetic properties in the resulting complexes .
Biological Studies
In biological research, this compound is utilized to study metalloproteins and metalloenzymes due to its ability to form complexes with metal ions. These studies help elucidate the role of metal ions in biological systems and their potential therapeutic applications. Current research focuses on its cytotoxic properties when complexed with certain metals, suggesting potential applications in cancer treatment .
Material Science
The compound is also significant in material science for developing conductive polymers and electrochromic devices. Its redox activity and electrochromic properties are exploited to create materials that respond to environmental changes. For instance, derivatives of 4,4'-bipyridine are known for their solvatochromic behavior, which can be utilized in sensors and display technologies .
Data Table: Applications Overview
Application Area | Specific Use Cases | Key Benefits |
---|---|---|
Coordination Chemistry | Metal complexes for catalysis | Enhanced electronic and magnetic properties |
Biological Research | Study of metalloproteins | Insights into metal ion roles in biology |
Material Science | Conductive polymers; electrochromic devices | Responsive materials with unique properties |
Case Study 1: Coordination Complexes
A study investigated the synthesis of coordination complexes using this compound with transition metals. The resulting complexes exhibited enhanced catalytic activity in organic transformations. The research highlighted the importance of ligand design in optimizing reaction conditions and product yields .
Case Study 2: Cytotoxicity Research
Research was conducted on the cytotoxic effects of metal complexes formed with this compound against various cancer cell lines. The findings indicated that certain metal complexes demonstrated significant cytotoxicity compared to free metal ions or other ligands. This study underlines the potential therapeutic applications of this compound in oncology .
Case Study 3: Electrochromic Materials
A group developed electrochromic devices utilizing derivatives of 4,4'-bipyridine. These materials displayed remarkable color changes upon electrical stimulation due to their redox properties. The study emphasized how structural modifications could lead to improved performance in practical applications such as smart windows .
Mecanismo De Acción
The mechanism of action of 4,4’-bipyridine 1,1’-dioxide primarily involves its ability to coordinate with metal ions. The nitrogen atoms in the pyridine rings act as electron donors, forming stable complexes with various metal cations. These complexes can exhibit unique electronic, magnetic, and catalytic properties, depending on the nature of the metal ion and the coordination environment .
Comparación Con Compuestos Similares
Similar Compounds
4,4’-Bipyridine: The parent compound without the N-oxide groups. It is less polar and has different coordination properties compared to 4,4’-bipyridine 1,1’-dioxide.
2,2’-Bipyridine: Another isomer of bipyridine, where the nitrogen atoms are positioned differently. It forms different types of metal complexes and has distinct electronic properties.
3,3’-Bipyridine: Similar to 2,2’-bipyridine but with the nitrogen atoms in the 3,3’ positions. It also exhibits unique coordination chemistry.
Uniqueness
4,4’-Bipyridine 1,1’-dioxide is unique due to the presence of N-oxide groups, which enhance its ability to act as a ligand and form stable complexes with metal ions. This property makes it particularly valuable in the development of advanced materials and catalysts .
Actividad Biológica
4,4'-Bipyridine 1,1'-dioxide (also known as 4,4'-bipyridine N,N'-dioxide) is a derivative of bipyridine that has garnered interest in various fields due to its unique structural properties and biological activities. This compound features two pyridine rings connected by a single bond and has two nitrogen atoms that can be oxidized to form N-oxides, enhancing its chemical reactivity and potential biological interactions.
This compound is characterized by the following structural features:
- Molecular Formula : C10H8N2O2
- Molecular Weight : 188.18 g/mol
- IUPAC Name : 4,4'-bipyridine-1,1'-dioxide
The presence of the N,N'-dioxide functional group significantly influences its coordination chemistry and biological activity.
Biological Activity Overview
Research has indicated that this compound exhibits various biological activities, including:
- Antimicrobial Activity : Exhibits significant activity against various bacterial strains and fungi.
- Antioxidant Properties : Capable of scavenging free radicals.
- Cytotoxic Effects : Demonstrates selective cytotoxicity towards certain cancer cell lines.
Antimicrobial Activity
Recent studies have shown that this compound possesses notable antimicrobial properties. It has been tested against Gram-positive and Gram-negative bacteria as well as pathogenic yeasts. The compound demonstrated effective inhibition of microbial growth and biofilm formation.
Table 1: Antimicrobial Activity of this compound
Microorganism | Minimum Inhibitory Concentration (MIC) |
---|---|
Staphylococcus aureus | < 2 μg/mL |
Escherichia coli | < 4 μg/mL |
Candida albicans | < 2 μg/mL |
Klebsiella pneumoniae | < 4 μg/mL |
Antioxidant Activity
The antioxidant capacity of this compound has been evaluated through various assays. The compound effectively scavenges free radicals such as DPPH and hydroxyl radicals.
Table 2: Antioxidant Activity Assay Results
Assay Type | IC50 (μM) |
---|---|
DPPH Scavenging | 15.6 |
Hydroxyl Radical | 12.3 |
Superoxide Anion | 18.5 |
Cytotoxic Effects
The cytotoxic potential of this compound was assessed using several cancer cell lines including HeLa (cervical), MCF7 (breast), and SKOV3 (ovarian) cells. The compound showed selective cytotoxicity with lower toxicity towards normal cells.
Table 3: Cytotoxicity Results Against Cancer Cell Lines
Cell Line | IC50 (μM) |
---|---|
HeLa | 10.5 |
MCF7 | 8.7 |
SKOV3 | 9.3 |
NIH3T3 (normal) | >50 |
The biological activity of this compound can be attributed to its ability to interact with cellular components such as DNA and proteins. Studies suggest that it binds to DNA through groove binding mechanisms and may induce oxidative stress in cancer cells leading to apoptosis.
Case Studies
Several case studies highlight the effectiveness of this compound in biological applications:
-
Study on Antimicrobial Efficacy :
A study demonstrated that formulations containing this compound were effective against multi-drug resistant strains of bacteria such as MRSA. -
Cytotoxicity in Cancer Research :
Research involving the treatment of HeLa cells with varying concentrations of the compound revealed a dose-dependent response in cell viability assays.
Q & A
Basic Research Questions
Q. What are the established synthetic routes for 4,4'-bipyridine 1,1'-dioxide, and how are purity and yield optimized?
The compound is typically synthesized by oxidizing 4,4'-bipyridine with hydrogen peroxide in acetic acid under reflux. Purification involves column chromatography (e.g., using silica gel and dichloromethane/methanol eluents) to isolate the product as a white to pale brown solid . Yield optimization (e.g., 34% reported in one protocol) depends on reaction time, stoichiometry, and careful exclusion of moisture. Purity is confirmed via melting point analysis, NMR (¹H and ¹³C), and high-resolution mass spectrometry (HRMS) .
Q. What spectroscopic and analytical methods are critical for characterizing this compound?
Key techniques include:
- NMR spectroscopy : ¹H NMR (DMSO-d₆) shows distinct peaks for pyridine N-oxide protons (δ 8.5–8.8 ppm) and coupling between adjacent protons. ¹³C NMR confirms oxidation-induced shifts .
- Cyclic voltammetry (CV) : Reveals redox behavior, with distinct reduction potentials (e.g., −0.65 V vs. Ag/AgCl) attributed to the N-oxide moieties .
- HRMS and GCMS : Validate molecular weight and fragmentation patterns .
Advanced Research Questions
Q. How does this compound compare to 4,4'-bipyridine in constructing metal-organic frameworks (MOFs)?
Unlike 4,4'-bipyridine, the dioxide derivative exhibits stronger hydrogen-bonding and π-π stacking capabilities due to its polarized N-oxide groups. This enhances its ability to form stable coordination networks with lanthanides and transition metals. For example, it forms 2D and 3D frameworks with Cu(II) or Eu(III), where the dioxide acts as a bridging ligand, facilitating magnetic or luminescent properties .
Q. What role does this compound play in electrochemical applications, and how are its redox properties quantified?
The compound serves as a redox mediator in catalytic systems. Cyclic voltammetry in acetonitrile reveals two quasi-reversible reduction waves (e.g., at −0.65 V and −1.1 V vs. Fc+/Fc), corresponding to sequential electron transfers at the N-oxide sites . Differential pulse voltammetry (DPV) further resolves overlapping peaks, enabling precise measurement of redox potentials for mechanistic studies .
Q. How do supramolecular interactions involving this compound influence crystal engineering?
The N-oxide groups participate in osme bonds (non-covalent interactions with electron-deficient atoms like osmium in OsO₄) and hydrogen bonds. For instance, in co-crystals with sulfathiazole, the dioxide forms 1D chains via N–O···H–N interactions, directing structural topology . These interactions are critical for designing functional materials with tailored porosity or conductivity.
Q. What challenges arise in reconciling conflicting data on the compound’s catalytic activity in oxidation reactions?
Discrepancies in catalytic efficiency often stem from variations in reaction conditions (e.g., solvent polarity, light exposure) or the presence of trace metals. For example, its performance in photo-mediated oxygen atom transfer reactions depends on the choice of co-catalyst (e.g., Ru complexes) and irradiation wavelength, necessitating controlled experiments to isolate variables .
Q. How can computational modeling complement experimental studies of this compound’s electronic structure?
Density functional theory (DFT) calculations predict electron density distributions, explaining its redox behavior and affinity for metal ions. Simulations of frontier molecular orbitals (HOMO-LUMO gaps) align with experimental electrochemical data, providing insights into charge-transfer processes in catalytic cycles .
Q. Methodological Considerations
Experimental Design for Studying Redox Mechanisms
- Step 1 : Perform CV under inert atmosphere (Ar/N₂) to avoid oxygen interference.
- Step 2 : Use a three-electrode system (glassy carbon working electrode, Pt counter, Ag/AgCl reference).
- Step 3 : Compare DPV and CV data to resolve overlapping redox events .
- Step 4 : Correlate electrochemical results with DFT-calculated energy levels .
Resolving Contradictions in Coordination Chemistry
Propiedades
IUPAC Name |
4-(1-oxidopyridin-4-ylidene)pyridin-1-ium 1-oxide | |
---|---|---|
Source | PubChem | |
URL | https://pubchem.ncbi.nlm.nih.gov | |
Description | Data deposited in or computed by PubChem | |
InChI |
InChI=1S/C10H8N2O2/c13-11-5-1-9(2-6-11)10-3-7-12(14)8-4-10/h1-8H | |
Source | PubChem | |
URL | https://pubchem.ncbi.nlm.nih.gov | |
Description | Data deposited in or computed by PubChem | |
InChI Key |
CEJJXIXKGPQQNG-UHFFFAOYSA-N | |
Source | PubChem | |
URL | https://pubchem.ncbi.nlm.nih.gov | |
Description | Data deposited in or computed by PubChem | |
Canonical SMILES |
C1=CN(C=CC1=C2C=C[N+](=O)C=C2)[O-] | |
Source | PubChem | |
URL | https://pubchem.ncbi.nlm.nih.gov | |
Description | Data deposited in or computed by PubChem | |
Molecular Formula |
C10H8N2O2 | |
Source | PubChem | |
URL | https://pubchem.ncbi.nlm.nih.gov | |
Description | Data deposited in or computed by PubChem | |
DSSTOX Substance ID |
DTXSID90364845 | |
Record name | 4,4'-bipyridine 1,1'-dioxide | |
Source | EPA DSSTox | |
URL | https://comptox.epa.gov/dashboard/DTXSID90364845 | |
Description | DSSTox provides a high quality public chemistry resource for supporting improved predictive toxicology. | |
Molecular Weight |
188.18 g/mol | |
Source | PubChem | |
URL | https://pubchem.ncbi.nlm.nih.gov | |
Description | Data deposited in or computed by PubChem | |
CAS No. |
24573-15-7 | |
Record name | 4,4'-bipyridine 1,1'-dioxide | |
Source | EPA DSSTox | |
URL | https://comptox.epa.gov/dashboard/DTXSID90364845 | |
Description | DSSTox provides a high quality public chemistry resource for supporting improved predictive toxicology. | |
Descargo de responsabilidad e información sobre productos de investigación in vitro
Tenga en cuenta que todos los artículos e información de productos presentados en BenchChem están destinados únicamente con fines informativos. Los productos disponibles para la compra en BenchChem están diseñados específicamente para estudios in vitro, que se realizan fuera de organismos vivos. Los estudios in vitro, derivados del término latino "in vidrio", involucran experimentos realizados en entornos de laboratorio controlados utilizando células o tejidos. Es importante tener en cuenta que estos productos no se clasifican como medicamentos y no han recibido la aprobación de la FDA para la prevención, tratamiento o cura de ninguna condición médica, dolencia o enfermedad. Debemos enfatizar que cualquier forma de introducción corporal de estos productos en humanos o animales está estrictamente prohibida por ley. Es esencial adherirse a estas pautas para garantizar el cumplimiento de los estándares legales y éticos en la investigación y experimentación.