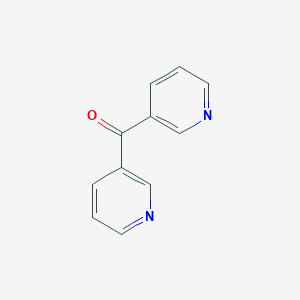
Di(piridin-3-il)metanona
Descripción general
Descripción
Di(pyridin-3-yl)methanone is an organic compound with the chemical formula C11H8N2O. It is characterized by the presence of two pyridine rings attached to a central methanone group. This compound appears as a white to pale yellow solid and is soluble in common organic solvents such as ethanol, methanol, and dimethylformamide. It is known for its stability under normal atmospheric conditions and its resistance to light and air degradation .
Aplicaciones Científicas De Investigación
Di(pyridin-3-yl)methanone has a wide range of applications in scientific research:
Métodos De Preparación
Synthetic Routes and Reaction Conditions: Di(pyridin-3-yl)methanone can be synthesized through several methods. One common approach involves the reaction of pyridine with benzyl bromide in the presence of a base. The reaction proceeds as follows:
- Benzyl bromide is added to a solution containing pyridine in an organic solvent.
- A base, such as sodium hydroxide or potassium carbonate, is introduced to the mixture.
- The reaction is allowed to proceed for a specified period, typically under reflux conditions.
- The product is then isolated and purified through a series of steps, including filtration and recrystallization .
Industrial Production Methods: In industrial settings, the production of di(pyridin-3-yl)methanone may involve more scalable and efficient processes. One such method is the copper-catalyzed oxidation of pyridin-2-yl-methanes using water as the oxygen source. This method offers a more environmentally friendly approach by avoiding the use of hazardous oxidants and operating under mild conditions .
Análisis De Reacciones Químicas
Types of Reactions: Di(pyridin-3-yl)methanone undergoes various chemical reactions, including:
Oxidation: The compound can be oxidized to form pyridin-2-yl-methanones.
Substitution: The methanone group can participate in substitution reactions, where different substituents replace the hydrogen atoms on the pyridine rings.
Common Reagents and Conditions:
Oxidation: Copper catalysts and water are commonly used for the oxidation of di(pyridin-3-yl)methanone.
Substitution: Various electrophiles and nucleophiles can be used in substitution reactions, depending on the desired product.
Major Products:
Oxidation: The major product of oxidation is pyridin-2-yl-methanone.
Substitution: The products vary based on the substituents introduced during the reaction.
Mecanismo De Acción
The mechanism of action of di(pyridin-3-yl)methanone involves its interaction with specific molecular targets and pathways. For instance, in oxidation reactions, the compound undergoes a copper-catalyzed process where water acts as the oxygen source. The reaction proceeds through a series of steps, including the formation of intermediates and the eventual production of the oxidized product .
Comparación Con Compuestos Similares
- Di(pyridin-2-yl)methanone
- Di(pyridin-4-yl)methanone
- Pyridin-3-yl (2-(2,3,4,5-tetra substituted phenyl)-1H-imidazol-1-yl) methanone
Comparison: Di(pyridin-3-yl)methanone is unique due to its specific structural arrangement, which imparts distinct chemical and physical properties. Compared to di(pyridin-2-yl)methanone and di(pyridin-4-yl)methanone, the 3-position substitution on the pyridine rings influences its reactivity and stability. Additionally, its applications in photochemical luminescent agents and metal complexes highlight its versatility and uniqueness .
Propiedades
IUPAC Name |
dipyridin-3-ylmethanone | |
---|---|---|
Source | PubChem | |
URL | https://pubchem.ncbi.nlm.nih.gov | |
Description | Data deposited in or computed by PubChem | |
InChI |
InChI=1S/C11H8N2O/c14-11(9-3-1-5-12-7-9)10-4-2-6-13-8-10/h1-8H | |
Source | PubChem | |
URL | https://pubchem.ncbi.nlm.nih.gov | |
Description | Data deposited in or computed by PubChem | |
InChI Key |
AQLPDLOXKZRZEV-UHFFFAOYSA-N | |
Source | PubChem | |
URL | https://pubchem.ncbi.nlm.nih.gov | |
Description | Data deposited in or computed by PubChem | |
Canonical SMILES |
C1=CC(=CN=C1)C(=O)C2=CN=CC=C2 | |
Source | PubChem | |
URL | https://pubchem.ncbi.nlm.nih.gov | |
Description | Data deposited in or computed by PubChem | |
Molecular Formula |
C11H8N2O | |
Source | PubChem | |
URL | https://pubchem.ncbi.nlm.nih.gov | |
Description | Data deposited in or computed by PubChem | |
DSSTOX Substance ID |
DTXSID80305388 | |
Record name | dipyridin-3-ylmethanone | |
Source | EPA DSSTox | |
URL | https://comptox.epa.gov/dashboard/DTXSID80305388 | |
Description | DSSTox provides a high quality public chemistry resource for supporting improved predictive toxicology. | |
Molecular Weight |
184.19 g/mol | |
Source | PubChem | |
URL | https://pubchem.ncbi.nlm.nih.gov | |
Description | Data deposited in or computed by PubChem | |
CAS No. |
35779-35-2 | |
Record name | 35779-35-2 | |
Source | DTP/NCI | |
URL | https://dtp.cancer.gov/dtpstandard/servlet/dwindex?searchtype=NSC&outputformat=html&searchlist=170628 | |
Description | The NCI Development Therapeutics Program (DTP) provides services and resources to the academic and private-sector research communities worldwide to facilitate the discovery and development of new cancer therapeutic agents. | |
Explanation | Unless otherwise indicated, all text within NCI products is free of copyright and may be reused without our permission. Credit the National Cancer Institute as the source. | |
Record name | dipyridin-3-ylmethanone | |
Source | EPA DSSTox | |
URL | https://comptox.epa.gov/dashboard/DTXSID80305388 | |
Description | DSSTox provides a high quality public chemistry resource for supporting improved predictive toxicology. | |
Record name | 35779-35-2 | |
Source | European Chemicals Agency (ECHA) | |
URL | https://echa.europa.eu/information-on-chemicals | |
Description | The European Chemicals Agency (ECHA) is an agency of the European Union which is the driving force among regulatory authorities in implementing the EU's groundbreaking chemicals legislation for the benefit of human health and the environment as well as for innovation and competitiveness. | |
Explanation | Use of the information, documents and data from the ECHA website is subject to the terms and conditions of this Legal Notice, and subject to other binding limitations provided for under applicable law, the information, documents and data made available on the ECHA website may be reproduced, distributed and/or used, totally or in part, for non-commercial purposes provided that ECHA is acknowledged as the source: "Source: European Chemicals Agency, http://echa.europa.eu/". Such acknowledgement must be included in each copy of the material. ECHA permits and encourages organisations and individuals to create links to the ECHA website under the following cumulative conditions: Links can only be made to webpages that provide a link to the Legal Notice page. | |
Synthesis routes and methods I
Procedure details
Synthesis routes and methods II
Procedure details
Retrosynthesis Analysis
AI-Powered Synthesis Planning: Our tool employs the Template_relevance Pistachio, Template_relevance Bkms_metabolic, Template_relevance Pistachio_ringbreaker, Template_relevance Reaxys, Template_relevance Reaxys_biocatalysis model, leveraging a vast database of chemical reactions to predict feasible synthetic routes.
One-Step Synthesis Focus: Specifically designed for one-step synthesis, it provides concise and direct routes for your target compounds, streamlining the synthesis process.
Accurate Predictions: Utilizing the extensive PISTACHIO, BKMS_METABOLIC, PISTACHIO_RINGBREAKER, REAXYS, REAXYS_BIOCATALYSIS database, our tool offers high-accuracy predictions, reflecting the latest in chemical research and data.
Strategy Settings
Precursor scoring | Relevance Heuristic |
---|---|
Min. plausibility | 0.01 |
Model | Template_relevance |
Template Set | Pistachio/Bkms_metabolic/Pistachio_ringbreaker/Reaxys/Reaxys_biocatalysis |
Top-N result to add to graph | 6 |
Feasible Synthetic Routes
Q1: What makes di(pyridin-3-yl)methanone a suitable acceptor unit in TADF emitters?
A1: Di(pyridin-3-yl)methanone, when coupled with appropriate donor units like carbazole derivatives, contributes to a small singlet-triplet energy gap (ΔEST) in the resulting TADF molecule [, ]. This small ΔEST is crucial for efficient TADF, allowing for up-conversion of triplet excitons to the singlet state and ultimately enhancing device efficiency. Both research papers demonstrate this effect, reporting ΔEST values as low as 0.01 eV for compounds incorporating di(pyridin-3-yl)methanone [, ].
Q2: How does the structure of the donor unit coupled with di(pyridin-3-yl)methanone impact the performance of the TADF emitter?
A2: The choice of donor unit significantly influences the performance of the TADF emitter. For instance, using 9,10-dihydro-9,9-dimethylacridine or phenoxazine modified carbazole as donors, coupled with di(pyridin-3-yl)methanone, resulted in different maximum luminance and current efficiency values in solution-processed devices []. This suggests that modifications to the donor structure affect the overall optoelectronic properties and device performance, likely by influencing factors like energy levels and charge transport.
Descargo de responsabilidad e información sobre productos de investigación in vitro
Tenga en cuenta que todos los artículos e información de productos presentados en BenchChem están destinados únicamente con fines informativos. Los productos disponibles para la compra en BenchChem están diseñados específicamente para estudios in vitro, que se realizan fuera de organismos vivos. Los estudios in vitro, derivados del término latino "in vidrio", involucran experimentos realizados en entornos de laboratorio controlados utilizando células o tejidos. Es importante tener en cuenta que estos productos no se clasifican como medicamentos y no han recibido la aprobación de la FDA para la prevención, tratamiento o cura de ninguna condición médica, dolencia o enfermedad. Debemos enfatizar que cualquier forma de introducción corporal de estos productos en humanos o animales está estrictamente prohibida por ley. Es esencial adherirse a estas pautas para garantizar el cumplimiento de los estándares legales y éticos en la investigación y experimentación.