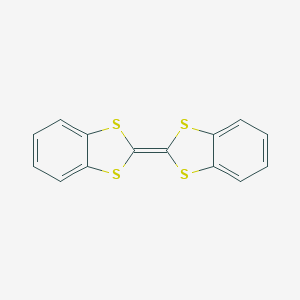
Dibenzotetrathiafulvalene
Descripción general
Descripción
Dibenzotetrathiafulvalene (DBTTF) is an organic semiconductor that is completely conjugated with a symmetric structure. It forms stacks of planar molecules with a distance of 3.948 Å .
Synthesis Analysis
DBTTF can be prepared from anthranilic acid and purified by sublimating in vacuum . A two-step synthesis of DBTTF derivatives has been developed by combining the indium-catalyzed reductive dithioacetalization of oxalic acid and electron-rich aromatic dithiols with a subsequent oxidation of the resultant dithioacetals .Molecular Structure Analysis
DBTTF has a high mobility of charges . Time-dependent density functional theory was used to explore the excitation and deexcitation processes .Chemical Reactions Analysis
DBTTF is used to make semiconducting charge transfer salts with electron accepting (n-type) materials, for example TCNQ and F4TCNQ . It forms a charge transferring complex with a variety of semiconducting crystals which include 7,7,8,8-tetracyanoquinodimethane (TCNQ) and 2,3-dichloro-5,6-dicyanobenzoquinone (DDQ) .Physical And Chemical Properties Analysis
DBTTF is a solid with a melting point of 239-243 °C . Its empirical formula is C14H8S4 and it has a molecular weight of 304.47 .Aplicaciones Científicas De Investigación
Photothermal Conversion
DBTTF has been used in the study of photothermal conversion mechanisms . The DBTTF-tetracyanobenzene (TCNB) cocrystal has shown higher photothermal conversion efficiency than its component single crystals . The high proportion of excited states promotes the occurrence of nonradiative transition, which might be the reason for the higher photothermal conversion efficiency .
Photothermal Imaging
DBTTF is used in photothermal imaging due to its photothermal conversion property . The unique photothermal properties of organic cocrystals like DBTTF make them suitable for this application .
Biological Applications
DBTTF has potential applications in the biological field . The photothermal properties of DBTTF can be utilized in various biological applications .
Seawater Desalination
DBTTF has been studied for its potential use in seawater desalination . The photothermal conversion property of DBTTF can be harnessed for this purpose .
Organic Electric Devices
DBTTF has been extensively studied for its applications in organic electric devices . Its properties such as metallic conductivity, photoconductivity, ambipolar charge transport, and high career mobility make it suitable for use in organic field effect transistors and solar cells .
Semiconducting Charge Transfer Salts
DBTTF is used to make semiconducting charge transfer salts with electron accepting (n-type) materials . This is particularly useful in the field of electronics .
Safety And Hazards
Direcciones Futuras
DBTTF-TCNB cocrystal has been bringing hope and vitality into several fields, such as photothermal imaging, biological application, and seawater desalination, owing to their photothermal conversion property . The results of the study may offer some guidance for the design of photothermal conversion cocrystals .
Propiedades
IUPAC Name |
2-(1,3-benzodithiol-2-ylidene)-1,3-benzodithiole | |
---|---|---|
Source | PubChem | |
URL | https://pubchem.ncbi.nlm.nih.gov | |
Description | Data deposited in or computed by PubChem | |
InChI |
InChI=1S/C14H8S4/c1-2-6-10-9(5-1)15-13(16-10)14-17-11-7-3-4-8-12(11)18-14/h1-8H | |
Source | PubChem | |
URL | https://pubchem.ncbi.nlm.nih.gov | |
Description | Data deposited in or computed by PubChem | |
InChI Key |
OVIRUXIWCFZJQC-UHFFFAOYSA-N | |
Source | PubChem | |
URL | https://pubchem.ncbi.nlm.nih.gov | |
Description | Data deposited in or computed by PubChem | |
Canonical SMILES |
C1=CC=C2C(=C1)SC(=C3SC4=CC=CC=C4S3)S2 | |
Source | PubChem | |
URL | https://pubchem.ncbi.nlm.nih.gov | |
Description | Data deposited in or computed by PubChem | |
Molecular Formula |
C14H8S4 | |
Source | PubChem | |
URL | https://pubchem.ncbi.nlm.nih.gov | |
Description | Data deposited in or computed by PubChem | |
DSSTOX Substance ID |
DTXSID20179388 | |
Record name | 1,3-Benzodithiole, 2-(1,3-benzodithiol-2-ylidene)- | |
Source | EPA DSSTox | |
URL | https://comptox.epa.gov/dashboard/DTXSID20179388 | |
Description | DSSTox provides a high quality public chemistry resource for supporting improved predictive toxicology. | |
Molecular Weight |
304.5 g/mol | |
Source | PubChem | |
URL | https://pubchem.ncbi.nlm.nih.gov | |
Description | Data deposited in or computed by PubChem | |
Product Name |
1,3-Benzodithiole, 2-(1,3-benzodithiol-2-ylidene)- | |
CAS RN |
24648-13-3 | |
Record name | Dibenzotetrathiafulvalene | |
Source | ChemIDplus | |
URL | https://pubchem.ncbi.nlm.nih.gov/substance/?source=chemidplus&sourceid=0024648133 | |
Description | ChemIDplus is a free, web search system that provides access to the structure and nomenclature authority files used for the identification of chemical substances cited in National Library of Medicine (NLM) databases, including the TOXNET system. | |
Record name | 1,3-Benzodithiole, 2-(1,3-benzodithiol-2-ylidene)- | |
Source | EPA DSSTox | |
URL | https://comptox.epa.gov/dashboard/DTXSID20179388 | |
Description | DSSTox provides a high quality public chemistry resource for supporting improved predictive toxicology. | |
Record name | 1,3-Benzodithiole, 2-(1,3-benzodithiol-2-ylidene)- | |
Source | European Chemicals Agency (ECHA) | |
URL | https://echa.europa.eu/information-on-chemicals | |
Description | The European Chemicals Agency (ECHA) is an agency of the European Union which is the driving force among regulatory authorities in implementing the EU's groundbreaking chemicals legislation for the benefit of human health and the environment as well as for innovation and competitiveness. | |
Explanation | Use of the information, documents and data from the ECHA website is subject to the terms and conditions of this Legal Notice, and subject to other binding limitations provided for under applicable law, the information, documents and data made available on the ECHA website may be reproduced, distributed and/or used, totally or in part, for non-commercial purposes provided that ECHA is acknowledged as the source: "Source: European Chemicals Agency, http://echa.europa.eu/". Such acknowledgement must be included in each copy of the material. ECHA permits and encourages organisations and individuals to create links to the ECHA website under the following cumulative conditions: Links can only be made to webpages that provide a link to the Legal Notice page. | |
Retrosynthesis Analysis
AI-Powered Synthesis Planning: Our tool employs the Template_relevance Pistachio, Template_relevance Bkms_metabolic, Template_relevance Pistachio_ringbreaker, Template_relevance Reaxys, Template_relevance Reaxys_biocatalysis model, leveraging a vast database of chemical reactions to predict feasible synthetic routes.
One-Step Synthesis Focus: Specifically designed for one-step synthesis, it provides concise and direct routes for your target compounds, streamlining the synthesis process.
Accurate Predictions: Utilizing the extensive PISTACHIO, BKMS_METABOLIC, PISTACHIO_RINGBREAKER, REAXYS, REAXYS_BIOCATALYSIS database, our tool offers high-accuracy predictions, reflecting the latest in chemical research and data.
Strategy Settings
Precursor scoring | Relevance Heuristic |
---|---|
Min. plausibility | 0.01 |
Model | Template_relevance |
Template Set | Pistachio/Bkms_metabolic/Pistachio_ringbreaker/Reaxys/Reaxys_biocatalysis |
Top-N result to add to graph | 6 |
Feasible Synthetic Routes
Q & A
Q1: What is the molecular formula and weight of DBTTF?
A1: The molecular formula of Dibenzotetrathiafulvalene is C14H8S4, and its molecular weight is 296.44 g/mol.
Q2: Is there spectroscopic data available for DBTTF?
A: Yes, DBTTF has been characterized by various spectroscopic techniques, including UV-Vis absorption spectroscopy, infrared (IR) spectroscopy, Raman spectroscopy, and X-ray photoelectron spectroscopy (XPS). [, , , , , , ] These techniques provide insights into its electronic structure, vibrational modes, and elemental composition.
Q3: What are the key structural features of DBTTF?
A: DBTTF is a planar molecule composed of a central tetrathiafulvalene (TTF) core fused with two benzene rings. This extended π-conjugated system contributes to its semiconducting properties. [, , ]
Q4: How many polymorphs of DBTTF have been identified and what are their characteristics?
A: Research has identified four polymorphic modifications of DBTTF at ambient conditions. These include a previously known monoclinic structure (α) with Z = 2, a second monoclinic structure (β) with Z = 4, and two additional polymorphs (γ and δ) characterized by X-ray diffraction and Raman microscopy. [] These variations in crystal structure impact the compound's physical and electronic properties.
Q5: How does the morphology of DBTTF thin films affect its performance in organic thin-film transistors (OTFTs)?
A: Controlling the film morphology of DBTTF is crucial for its performance in OTFTs. Studies have demonstrated that surface treatments on silicon dioxide dielectric substrates can tune the grain size of DBTTF polycrystalline films. This directly impacts the field-effect mobility and subthreshold characteristics of the transistors. [, ]
Q6: Does DBTTF exhibit polymorphism in its charge-transfer complexes?
A: Yes, DBTTF forms charge-transfer complexes with acceptors like 7,7,8,8-tetracyanoquinodimethane (TCNQ), and these complexes can exist in different polymorphic forms. For instance, DBTTF-TCNQ has been found to crystallize in two distinct polymorphs, α and β. [, ]
Q7: How does polymorphism in DBTTF-TCNQ affect its properties?
A: The different polymorphic forms of DBTTF-TCNQ exhibit distinct optical and electronic properties. Variations in donor-acceptor overlap and frontier molecular orbital mixing contribute to these differences. For example, the α-polymorph of DBTTF-TCNQ exhibits electron-dominant transport, while the β-polymorph shows hole-dominant transport in organic field-effect transistors (OFETs). []
Q8: How does the degree of charge transfer in DBTTF-TCNQ impact its properties?
A: The degree of charge transfer between DBTTF and TCNQ significantly affects the complex's properties. For instance, the α-polymorph of DBTTF-TCNQ with a higher degree of charge transfer (≈0.5e) exhibits different optical and electronic properties compared to the nearly neutral β-polymorph. []
Q9: What are the potential applications of DBTTF in organic electronics?
A: DBTTF and its derivatives are being explored for applications in organic field-effect transistors (OFETs) [, , , ], solar cells [, ], and other organic electronic devices [, , , ]. Its semiconducting properties, ability to form charge-transfer complexes, and potential for solution processing make it a promising material in this field.
Q10: How does DBTTF interact with electron acceptors?
A: DBTTF acts as an electron donor and readily forms charge-transfer complexes with electron acceptors such as TCNQ, 2,3-dichloro-5,6-dicyano-p-benzoquinone (DDQ), and fullerene (C60). [, , , , , ] These interactions lead to unique optical and electronic properties.
Q11: What is the significance of the interaction between DBTTF and C60 in materials science?
A: The interaction between DBTTF and C60 leads to the formation of charge-transfer complexes with intriguing optoelectronic properties. For instance, nanosheets composed of DBTTF and C60 exhibit a significant photostrictive effect, meaning they undergo mechanical deformation upon light exposure. This property makes them promising for applications in photoswitchable devices and flexible electronics. []
Q12: What is the role of DBTTF in the formation of conductive charge-transfer complexes?
A: DBTTF acts as an electron donor in charge-transfer complexes. The degree of charge transfer between DBTTF and the acceptor molecule significantly influences the complex's conductivity. Complexes with partial charge transfer often exhibit high conductivity. [, , ] For example, DBTTF-DDQ is a highly conducting charge-transfer salt with a room temperature pellet conductivity of 8 Ω−1 cm−1. []
Q13: How does the molecular structure of DBTTF influence its ability to form conductive complexes?
A: The extended π-conjugated system and the presence of sulfur atoms in DBTTF's structure are crucial for its electron-donating ability and its tendency to form conductive complexes. [, ] The sulfur atoms can participate in intermolecular interactions, further influencing the packing and electronic properties of the complexes.
Q14: How does DBTTF interact with metal ions or complexes?
A: DBTTF can interact with metal ions or complexes, forming salts with distinct crystal structures and properties. For instance, DBTTF forms a salt with a chloride-bridged trimerized dimethyltin(IV) dianion, resulting in a columnar structure of DBTTF units. []
Q15: Can DBTTF form supramolecular structures?
A: Yes, DBTTF has been shown to form supramolecular networks. For example, the oxidation of DBTTF in the presence of Keggin-type polyoxoanions leads to the formation of a three-dimensional supramolecular network with one-dimensional channels. These channels are occupied by uncoordinated water molecules. []
Q16: Have there been computational studies on DBTTF and its derivatives?
A: Yes, computational chemistry techniques, including Density Functional Theory (DFT) calculations, have been employed to study the electronic structure, charge transfer properties, and molecular packing of DBTTF and its complexes. [, , ] These studies provide valuable insights into the relationship between molecular structure and material properties.
Q17: What are the future directions for research on DBTTF?
A17: Future research on DBTTF is likely to focus on:
- Developing new derivatives with tailored properties: This includes exploring different substituents, heteroatom substitutions, and extended π-conjugated systems. [, , ]
- Optimizing processing techniques: Efforts will focus on achieving controlled thin-film morphology and developing scalable deposition methods. [, ]
- Deeper understanding of charge transfer mechanisms: This involves investigating the factors influencing charge transfer efficiency and developing strategies to enhance conductivity in DBTTF-based materials. [, ]
- Exploring novel applications: This includes investigating DBTTF's potential in areas like sensors, photovoltaics, and spintronics. [, , ]
Q18: What is known about the stability and degradation of DBTTF?
A: While DBTTF itself is relatively stable under ambient conditions, its stability can be influenced by factors such as exposure to air, light, and temperature. [, ] Research on the degradation pathways of DBTTF and its derivatives is crucial for understanding their long-term performance in various applications.
Q19: Are there any environmental concerns regarding DBTTF and its derivatives?
A: As with any new material, understanding the environmental impact of DBTTF and its derivatives is essential. Research on their ecotoxicological effects, biodegradability, and potential for recycling or safe disposal is crucial for ensuring their sustainable use. [, ]
Descargo de responsabilidad e información sobre productos de investigación in vitro
Tenga en cuenta que todos los artículos e información de productos presentados en BenchChem están destinados únicamente con fines informativos. Los productos disponibles para la compra en BenchChem están diseñados específicamente para estudios in vitro, que se realizan fuera de organismos vivos. Los estudios in vitro, derivados del término latino "in vidrio", involucran experimentos realizados en entornos de laboratorio controlados utilizando células o tejidos. Es importante tener en cuenta que estos productos no se clasifican como medicamentos y no han recibido la aprobación de la FDA para la prevención, tratamiento o cura de ninguna condición médica, dolencia o enfermedad. Debemos enfatizar que cualquier forma de introducción corporal de estos productos en humanos o animales está estrictamente prohibida por ley. Es esencial adherirse a estas pautas para garantizar el cumplimiento de los estándares legales y éticos en la investigación y experimentación.