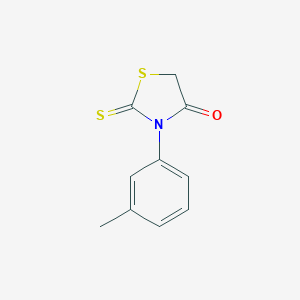
2-Thioxo-3-m-tolyl-thiazolidin-4-one
Descripción general
Descripción
2-Thioxo-3-m-tolyl-thiazolidin-4-one is a heterocyclic compound that belongs to the rhodanine family. Rhodanine derivatives are known for their diverse biological activities and have been extensively studied for their potential applications in medicinal chemistry. The compound features a thiazolidine ring with a thioxo group at the second carbon and a carbonyl group at the fourth carbon, making it a versatile scaffold for various chemical modifications.
Mecanismo De Acción
Target of Action
Rhodanine derivatives have been found to exhibit potent anticancer activity . They have been shown to inhibit protein tyrosine phosphatase type IVA, member 3 (PRL-3) enzymatic activity . PRL-3 is overexpressed in various types of cancer cells, and its inhibition can lead to reduced migration and invasion of these cells .
Mode of Action
The mode of action of Rhodanine, 3-(m-tolyl)-, involves interaction with its targets, leading to changes in cellular processes. The compound is electrophilic and potentially reactive due to the exocyclic double bond conjugated to the carbonyl group at position 4 of the rhodanine ring . This allows for possible Michael addition of the nucleophilic protein residues to the exocyclic double bond .
Biochemical Pathways
The inhibition of prl-3 enzymatic activity suggests that it may impact pathways related to cell migration and invasion
Result of Action
The result of Rhodanine, 3-(m-tolyl)-'s action is the inhibition of cancer cell migration and invasion . This is due to its inhibitory effect on PRL-3 enzymatic activity . The compound has shown potent anticancer activity against diverse cancer cell lines .
Análisis Bioquímico
Biochemical Properties
Rhodanine, 3-(m-tolyl)- plays a crucial role in biochemical reactions by interacting with enzymes, proteins, and other biomolecules. It has been shown to inhibit the activity of certain enzymes, such as protein tyrosine phosphatase type IVA, member 3 (PRL-3), which is involved in cancer cell migration and invasion . Additionally, Rhodanine, 3-(m-tolyl)- interacts with aldose reductase and aldehyde reductase, enzymes involved in the polyol pathway, which is significant in managing diabetic complications . These interactions highlight the compound’s potential as a therapeutic agent.
Cellular Effects
Rhodanine, 3-(m-tolyl)- exhibits various effects on different cell types and cellular processes. It has demonstrated potent anticancer activity against several cancer cell lines, including HeLa (cervical carcinoma), MCF-7 (human breast cancer), and HT-29 (colon cancer) cells . The compound influences cell function by modulating cell signaling pathways, gene expression, and cellular metabolism. For instance, it has been shown to inhibit the migration and invasion of cancer cells by targeting specific signaling pathways .
Molecular Mechanism
The molecular mechanism of Rhodanine, 3-(m-tolyl)- involves its binding interactions with biomolecules, enzyme inhibition, and changes in gene expression. The compound binds to the active sites of enzymes, such as PRL-3, leading to their inhibition . This inhibition disrupts the downstream signaling pathways, resulting in reduced cancer cell migration and invasion. Additionally, Rhodanine, 3-(m-tolyl)- can modulate gene expression by interacting with transcription factors and other regulatory proteins .
Temporal Effects in Laboratory Settings
In laboratory settings, the effects of Rhodanine, 3-(m-tolyl)- have been observed to change over time. The compound’s stability and degradation play a crucial role in its long-term effects on cellular function. Studies have shown that Rhodanine, 3-(m-tolyl)- remains stable under specific conditions, allowing for prolonged activity in in vitro and in vivo experiments . Its degradation products may exhibit different biological activities, which need to be considered in long-term studies .
Dosage Effects in Animal Models
The effects of Rhodanine, 3-(m-tolyl)- vary with different dosages in animal models. At lower doses, the compound has shown therapeutic benefits, such as reducing cancer cell proliferation and migration . At higher doses, it may exhibit toxic or adverse effects, including hepatotoxicity and nephrotoxicity . These dosage-dependent effects highlight the importance of optimizing the dosage for therapeutic applications.
Metabolic Pathways
Rhodanine, 3-(m-tolyl)- is involved in various metabolic pathways, interacting with enzymes and cofactors. It has been shown to inhibit aldose reductase and aldehyde reductase, key enzymes in the polyol pathway . This inhibition reduces the accumulation of sorbitol, a metabolite associated with diabetic complications. Additionally, Rhodanine, 3-(m-tolyl)- can modulate glucose metabolism by activating the peroxisome proliferator-activated receptor gamma (PPAR-γ), improving insulin sensitivity .
Transport and Distribution
The transport and distribution of Rhodanine, 3-(m-tolyl)- within cells and tissues involve interactions with transporters and binding proteins. The compound can be transported across cell membranes via specific transporters, allowing it to reach its target sites . Once inside the cells, Rhodanine, 3-(m-tolyl)- may bind to proteins that facilitate its localization and accumulation in specific cellular compartments .
Subcellular Localization
Rhodanine, 3-(m-tolyl)- exhibits specific subcellular localization, which influences its activity and function. The compound can be directed to specific compartments or organelles through targeting signals or post-translational modifications . For example, it may localize to the nucleus, where it interacts with transcription factors to modulate gene expression . Understanding the subcellular localization of Rhodanine, 3-(m-tolyl)- is essential for elucidating its precise mechanisms of action.
Métodos De Preparación
Synthetic Routes and Reaction Conditions
The synthesis of 2-Thioxo-3-m-tolyl-thiazolidin-4-one typically involves the condensation of rhodanine with m-tolyl aldehyde. A common method includes the reaction of rhodanine with m-tolyl aldehyde in the presence of a base such as sodium acetate in acetic acid under reflux conditions . The reaction mixture is heated for several hours until the completion of the reaction, which is monitored by thin-layer chromatography (TLC).
Industrial Production Methods
Industrial production methods for this compound are similar to laboratory synthesis but are scaled up to accommodate larger quantities. The process involves the use of industrial-grade reagents and solvents, and the reaction is carried out in large reactors with efficient stirring and temperature control to ensure consistent product quality.
Análisis De Reacciones Químicas
Types of Reactions
2-Thioxo-3-m-tolyl-thiazolidin-4-one undergoes various chemical reactions, including:
Oxidation: The compound can be oxidized to form sulfoxides or sulfones.
Reduction: Reduction reactions can convert the carbonyl group to a hydroxyl group.
Substitution: The thiazolidine ring allows for nucleophilic substitution reactions, where different substituents can be introduced at various positions on the ring.
Common Reagents and Conditions
Oxidation: Common oxidizing agents include hydrogen peroxide and m-chloroperbenzoic acid.
Reduction: Reducing agents such as sodium borohydride or lithium aluminum hydride are used.
Substitution: Nucleophiles like amines or thiols can be used in substitution reactions under basic or acidic conditions.
Major Products Formed
The major products formed from these reactions depend on the specific reagents and conditions used. For example, oxidation can yield sulfoxides or sulfones, while substitution reactions can introduce various functional groups, enhancing the compound’s biological activity.
Aplicaciones Científicas De Investigación
Chemistry: Used as a building block for synthesizing more complex molecules.
Biology: Investigated for its antimicrobial and antifungal properties.
Medicine: Explored for its potential as an anticancer and antidiabetic agent.
Industry: Utilized in the development of dyes and pigments due to its chromophoric properties.
Comparación Con Compuestos Similares
Similar Compounds
- Rhodanine-3-acetic acid
- Rhodanine-3-propionic acid
- Rhodanine-3-butyric acid
Comparison
2-Thioxo-3-m-tolyl-thiazolidin-4-one is unique due to the presence of the m-tolyl group, which enhances its biological activity compared to other rhodanine derivatives. The m-tolyl group provides additional hydrophobic interactions and steric effects, making it more effective in certain applications .
Propiedades
IUPAC Name |
3-(3-methylphenyl)-2-sulfanylidene-1,3-thiazolidin-4-one | |
---|---|---|
Source | PubChem | |
URL | https://pubchem.ncbi.nlm.nih.gov | |
Description | Data deposited in or computed by PubChem | |
InChI |
InChI=1S/C10H9NOS2/c1-7-3-2-4-8(5-7)11-9(12)6-14-10(11)13/h2-5H,6H2,1H3 | |
Source | PubChem | |
URL | https://pubchem.ncbi.nlm.nih.gov | |
Description | Data deposited in or computed by PubChem | |
InChI Key |
SWXZAZUMBRCACD-UHFFFAOYSA-N | |
Source | PubChem | |
URL | https://pubchem.ncbi.nlm.nih.gov | |
Description | Data deposited in or computed by PubChem | |
Canonical SMILES |
CC1=CC(=CC=C1)N2C(=O)CSC2=S | |
Source | PubChem | |
URL | https://pubchem.ncbi.nlm.nih.gov | |
Description | Data deposited in or computed by PubChem | |
Molecular Formula |
C10H9NOS2 | |
Source | PubChem | |
URL | https://pubchem.ncbi.nlm.nih.gov | |
Description | Data deposited in or computed by PubChem | |
DSSTOX Substance ID |
DTXSID60178113 | |
Record name | Rhodanine, 3-(m-tolyl)- | |
Source | EPA DSSTox | |
URL | https://comptox.epa.gov/dashboard/DTXSID60178113 | |
Description | DSSTox provides a high quality public chemistry resource for supporting improved predictive toxicology. | |
Molecular Weight |
223.3 g/mol | |
Source | PubChem | |
URL | https://pubchem.ncbi.nlm.nih.gov | |
Description | Data deposited in or computed by PubChem | |
CAS No. |
23522-38-5 | |
Record name | 3-m-Tolylrhodanine | |
Source | ChemIDplus | |
URL | https://pubchem.ncbi.nlm.nih.gov/substance/?source=chemidplus&sourceid=0023522385 | |
Description | ChemIDplus is a free, web search system that provides access to the structure and nomenclature authority files used for the identification of chemical substances cited in National Library of Medicine (NLM) databases, including the TOXNET system. | |
Record name | 23522-38-5 | |
Source | DTP/NCI | |
URL | https://dtp.cancer.gov/dtpstandard/servlet/dwindex?searchtype=NSC&outputformat=html&searchlist=32124 | |
Description | The NCI Development Therapeutics Program (DTP) provides services and resources to the academic and private-sector research communities worldwide to facilitate the discovery and development of new cancer therapeutic agents. | |
Explanation | Unless otherwise indicated, all text within NCI products is free of copyright and may be reused without our permission. Credit the National Cancer Institute as the source. | |
Record name | Rhodanine, 3-(m-tolyl)- | |
Source | EPA DSSTox | |
URL | https://comptox.epa.gov/dashboard/DTXSID60178113 | |
Description | DSSTox provides a high quality public chemistry resource for supporting improved predictive toxicology. | |
Record name | 3-M-TOLYLRHODANINE | |
Source | FDA Global Substance Registration System (GSRS) | |
URL | https://gsrs.ncats.nih.gov/ginas/app/beta/substances/N501N6TY7K | |
Description | The FDA Global Substance Registration System (GSRS) enables the efficient and accurate exchange of information on what substances are in regulated products. Instead of relying on names, which vary across regulatory domains, countries, and regions, the GSRS knowledge base makes it possible for substances to be defined by standardized, scientific descriptions. | |
Explanation | Unless otherwise noted, the contents of the FDA website (www.fda.gov), both text and graphics, are not copyrighted. They are in the public domain and may be republished, reprinted and otherwise used freely by anyone without the need to obtain permission from FDA. Credit to the U.S. Food and Drug Administration as the source is appreciated but not required. | |
Descargo de responsabilidad e información sobre productos de investigación in vitro
Tenga en cuenta que todos los artículos e información de productos presentados en BenchChem están destinados únicamente con fines informativos. Los productos disponibles para la compra en BenchChem están diseñados específicamente para estudios in vitro, que se realizan fuera de organismos vivos. Los estudios in vitro, derivados del término latino "in vidrio", involucran experimentos realizados en entornos de laboratorio controlados utilizando células o tejidos. Es importante tener en cuenta que estos productos no se clasifican como medicamentos y no han recibido la aprobación de la FDA para la prevención, tratamiento o cura de ninguna condición médica, dolencia o enfermedad. Debemos enfatizar que cualquier forma de introducción corporal de estos productos en humanos o animales está estrictamente prohibida por ley. Es esencial adherirse a estas pautas para garantizar el cumplimiento de los estándares legales y éticos en la investigación y experimentación.