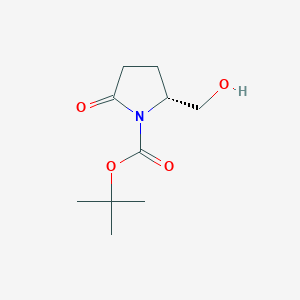
Boc-D-Pyroglutaminol
Descripción general
Descripción
Métodos De Preparación
Synthetic Routes and Reaction Conditions
The preparation of Boc-D-Pyroglutaminol typically involves the protection of pyroglutamic acid followed by reduction to obtain the corresponding amino alcohol compound . The general synthetic route includes:
Protection of Pyroglutamic Acid: Pyroglutamic acid is first protected using tert-butoxycarbonyl (Boc) groups to form Boc-pyroglutamic acid.
Industrial Production Methods
Industrial production methods for this compound are similar to laboratory synthesis but are scaled up to accommodate larger quantities. The process involves the same protection and reduction steps, with optimizations for yield and purity. The reaction conditions are carefully controlled to ensure consistent product quality .
Análisis De Reacciones Químicas
Types of Reactions
Boc-D-Pyroglutaminol undergoes various chemical reactions, including:
Oxidation: The compound can be oxidized to form corresponding oxo derivatives.
Reduction: Further reduction can lead to the formation of different amino alcohol derivatives.
Substitution: It can participate in substitution reactions where the Boc group is replaced by other functional groups.
Common Reagents and Conditions
Oxidation: Common oxidizing agents include potassium permanganate (KMnO4) and chromium trioxide (CrO3).
Reduction: Reducing agents such as lithium aluminum hydride (LiAlH4) and sodium borohydride (NaBH4) are used.
Substitution: Reagents like trifluoroacetic acid (TFA) are used to remove the Boc group.
Major Products Formed
Oxidation: Oxo derivatives of this compound.
Reduction: Various amino alcohol derivatives.
Substitution: Compounds with different functional groups replacing the Boc group.
Aplicaciones Científicas De Investigación
Scientific Research Applications
Boc-D-Pyroglutaminol serves multiple roles across different fields:
- Chemistry : It is primarily used as a chiral auxiliary in peptide synthesis. The compound facilitates the formation of peptide bonds with precise stereochemistry, essential for synthesizing complex peptides.
- Biology : The compound has been studied for its role in synthesizing biologically active peptides and proteins. Its ability to influence stereochemistry is crucial in creating functional peptides that can interact with biological targets.
- Medicine : Research indicates potential therapeutic effects, particularly in drug development aimed at treating various diseases. Its derivatives have shown promise in inhibiting specific enzymes and exhibiting antiviral activity.
- Industry : this compound is employed in producing complex peptides and proteins for industrial applications, including pharmaceuticals and biotechnology.
Research has highlighted several biological activities associated with this compound:
- Enzyme Inhibition : Derivatives have been found to inhibit glycosidases, which play critical roles in carbohydrate metabolism.
- Antiviral Activity : Studies indicate that certain derivatives exhibit anti-HIV and anti-HCV activity. For instance, one study reported an EC50 value of 36.9 μM against HIV-1, demonstrating moderate antiviral efficacy compared to established agents like AZT .
Case Study 1: Functionalization of Pyroglutaminols
A study conducted by Pfizer focused on the functionalization of protected pyroglutaminols to develop anti-inflammatory agents. The research highlighted how lithium enolates derived from this compound can be selectively functionalized for various applications .
Case Study 2: Antiviral Research
Another significant study evaluated the antiviral potential of this compound derivatives against HIV-1 and HCV. The findings suggested that these compounds could serve as a basis for developing new antiviral therapies .
Mecanismo De Acción
The mechanism of action of Boc-D-Pyroglutaminol is related to its role as a chiral auxiliary in peptide synthesis. It allows for the selective formation of peptide bonds in a stereocontrolled manner, which is crucial for the synthesis of complex peptide sequences. The compound interacts with molecular targets and pathways involved in peptide bond formation, ensuring the correct stereochemistry is achieved.
Comparación Con Compuestos Similares
Boc-D-Pyroglutaminol is unique compared to other similar compounds due to its specific role as a chiral auxiliary in peptide synthesis. Similar compounds include:
Boc-L-Pyroglutaminol: The L-enantiomer of this compound, used in similar applications but with different stereochemistry.
N-Cbz-Pyroglutaminol: Another protected form of pyroglutaminol, used in peptide synthesis with different protecting groups.
N-Methoxycarbonyl-Pyroglutaminol: Similar to this compound but with a different protecting group, used in peptide synthesis.
Actividad Biológica
Boc-D-Pyroglutaminol is a derivative of pyroglutamic acid, which has garnered attention in medicinal chemistry due to its potential biological activities. This article reviews the biological activity of this compound, focusing on its mechanisms of action, therapeutic applications, and relevant research findings.
Chemical Structure and Properties
This compound is characterized by a tert-butyloxycarbonyl (Boc) protecting group attached to the nitrogen atom of pyroglutaminol. This modification enhances its stability and solubility in biological systems, making it a valuable compound for drug development.
The biological activity of this compound can be attributed to several mechanisms:
- Inhibition of Enzymatic Activity : Research indicates that derivatives of pyroglutaminol can inhibit specific enzymes involved in various metabolic pathways. For instance, this compound has shown promise in inhibiting glycosidases, which are crucial for carbohydrate metabolism .
- Antiviral Activity : Studies have demonstrated that certain derivatives exhibit anti-HIV and anti-HCV activity. For example, compounds derived from pyroglutaminol have been evaluated for their efficacy against these viruses, showing varying degrees of effectiveness .
Antiviral Activity
The antiviral potential of this compound was evaluated in vitro against HIV-1 and HCV. In one study, the compound demonstrated an EC50 value of 36.9 μM against HIV-1, indicating moderate antiviral activity compared to established antiviral agents like AZT .
Cytotoxicity Studies
Cytotoxicity assays were performed on various cell lines to assess the safety profile of this compound. Most derivatives showed low toxicity levels, with significant cytotoxic effects observed only at higher concentrations in specific cell lines (e.g., Vero cells) . The results are summarized in Table 1.
Compound | Cell Line | EC50 (μM) | Toxicity Observed |
---|---|---|---|
This compound | CEM | 36.9 | Low |
This compound | Vero | 44.5 | Moderate |
Other Derivatives | PBM | Varies | Low |
Study 1: Functionalization and Selectivity
A study focused on the functionalization of this compound derivatives highlighted their selectivity in reactions involving lithium enolates. The research demonstrated that certain modifications could enhance selectivity for specific reactions, which is crucial for developing targeted therapeutics .
Study 2: Anti-inflammatory Properties
Another investigation explored the anti-inflammatory properties of this compound derivatives. The results indicated that these compounds could modulate inflammatory pathways, suggesting potential applications in treating inflammatory diseases .
Propiedades
IUPAC Name |
tert-butyl (2R)-2-(hydroxymethyl)-5-oxopyrrolidine-1-carboxylate | |
---|---|---|
Source | PubChem | |
URL | https://pubchem.ncbi.nlm.nih.gov | |
Description | Data deposited in or computed by PubChem | |
InChI |
InChI=1S/C10H17NO4/c1-10(2,3)15-9(14)11-7(6-12)4-5-8(11)13/h7,12H,4-6H2,1-3H3/t7-/m1/s1 | |
Source | PubChem | |
URL | https://pubchem.ncbi.nlm.nih.gov | |
Description | Data deposited in or computed by PubChem | |
InChI Key |
NYYVBJAPYGKIEN-SSDOTTSWSA-N | |
Source | PubChem | |
URL | https://pubchem.ncbi.nlm.nih.gov | |
Description | Data deposited in or computed by PubChem | |
Canonical SMILES |
CC(C)(C)OC(=O)N1C(CCC1=O)CO | |
Source | PubChem | |
URL | https://pubchem.ncbi.nlm.nih.gov | |
Description | Data deposited in or computed by PubChem | |
Isomeric SMILES |
CC(C)(C)OC(=O)N1[C@H](CCC1=O)CO | |
Source | PubChem | |
URL | https://pubchem.ncbi.nlm.nih.gov | |
Description | Data deposited in or computed by PubChem | |
Molecular Formula |
C10H17NO4 | |
Source | PubChem | |
URL | https://pubchem.ncbi.nlm.nih.gov | |
Description | Data deposited in or computed by PubChem | |
DSSTOX Substance ID |
DTXSID10526322 | |
Record name | tert-Butyl (2R)-2-(hydroxymethyl)-5-oxopyrrolidine-1-carboxylate | |
Source | EPA DSSTox | |
URL | https://comptox.epa.gov/dashboard/DTXSID10526322 | |
Description | DSSTox provides a high quality public chemistry resource for supporting improved predictive toxicology. | |
Molecular Weight |
215.25 g/mol | |
Source | PubChem | |
URL | https://pubchem.ncbi.nlm.nih.gov | |
Description | Data deposited in or computed by PubChem | |
CAS No. |
128811-37-0 | |
Record name | 1,1-Dimethylethyl (2R)-2-(hydroxymethyl)-5-oxo-1-pyrrolidinecarboxylate | |
Source | CAS Common Chemistry | |
URL | https://commonchemistry.cas.org/detail?cas_rn=128811-37-0 | |
Description | CAS Common Chemistry is an open community resource for accessing chemical information. Nearly 500,000 chemical substances from CAS REGISTRY cover areas of community interest, including common and frequently regulated chemicals, and those relevant to high school and undergraduate chemistry classes. This chemical information, curated by our expert scientists, is provided in alignment with our mission as a division of the American Chemical Society. | |
Explanation | The data from CAS Common Chemistry is provided under a CC-BY-NC 4.0 license, unless otherwise stated. | |
Record name | tert-Butyl (2R)-2-(hydroxymethyl)-5-oxopyrrolidine-1-carboxylate | |
Source | EPA DSSTox | |
URL | https://comptox.epa.gov/dashboard/DTXSID10526322 | |
Description | DSSTox provides a high quality public chemistry resource for supporting improved predictive toxicology. | |
Descargo de responsabilidad e información sobre productos de investigación in vitro
Tenga en cuenta que todos los artículos e información de productos presentados en BenchChem están destinados únicamente con fines informativos. Los productos disponibles para la compra en BenchChem están diseñados específicamente para estudios in vitro, que se realizan fuera de organismos vivos. Los estudios in vitro, derivados del término latino "in vidrio", involucran experimentos realizados en entornos de laboratorio controlados utilizando células o tejidos. Es importante tener en cuenta que estos productos no se clasifican como medicamentos y no han recibido la aprobación de la FDA para la prevención, tratamiento o cura de ninguna condición médica, dolencia o enfermedad. Debemos enfatizar que cualquier forma de introducción corporal de estos productos en humanos o animales está estrictamente prohibida por ley. Es esencial adherirse a estas pautas para garantizar el cumplimiento de los estándares legales y éticos en la investigación y experimentación.