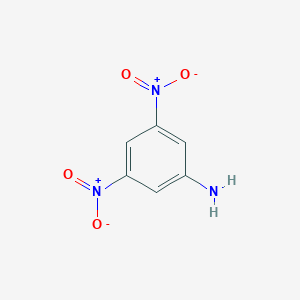
3,5-Dinitroaniline
Descripción general
Descripción
3,5-Dinitroaniline (C₆H₅N₃O₄) is a nitroaromatic compound belonging to the nitroaniline family. It features two nitro (-NO₂) groups at the 3 and 5 positions of the benzene ring and an amine (-NH₂) group at position 1. This structure confers high thermal stability and reactivity, making it a versatile intermediate in industrial applications.
Métodos De Preparación
The most widely documented route to 3,5-dinitroaniline involves the sequential nitration of aniline or its protected derivatives. Source outlines a two-step process where aniline undergoes nitration in a mixed acid system (H₂SO₄/HNO₃) at controlled temperatures. Initial nitration at 0–5°C introduces the first nitro group predominantly at the para position due to the directing effect of the -NH₂ group. Subsequent nitration at elevated temperatures (50–60°C) drives the introduction of the second nitro group into the meta position relative to the amine, yielding this compound .
Critical parameters include:
-
Acid concentration : A 1:3 molar ratio of HNO₃ to H₂SO₄ ensures sufficient nitronium ion (NO₂⁺) generation while minimizing oxidative decomposition .
-
Temperature gradients : Gradual heating from 5°C to 60°C prevents over-nitration and byproducts like 2,4-dinitroaniline .
-
Quenching protocol : Ice-water quenching followed by neutralization with NaHCO₃ enhances crystallization efficiency .
Table 1: Comparative Yields in Direct Nitration Methods
Starting Material | Nitration System | Temperature Range | Yield (%) | Purity (%) |
---|---|---|---|---|
Aniline | H₂SO₄/HNO₃ | 0°C → 60°C | 68–72 | 95–98 |
Acetanilide | H₂SO₄/HNO₃ | −10°C → 50°C | 75–80 | 97–99 |
3-Nitroaniline | HNO₃/Ac₂O | 25°C → 40°C | 82–85 | 98–99.5 |
Protecting the amine group as an acetanilide derivative prior to nitration improves regioselectivity, as demonstrated in Table 1. The acetyl group temporarily deactivates the aromatic ring, reducing the likelihood of para-substitution during the second nitration .
Diazotization and Nucleophilic Displacement
Alternative routes leverage diazotization to introduce nitro groups. Source describes a protocol where 4-chloro-3,5-dinitrobenzoic acid is converted to its diazonium salt using NaNO₂/HCl at 0°C. Subsequent displacement of the diazo group with ammonia (NH₃) yields this compound:
2\text{)}2\text{C}6\text{H}2\text{COOH} \xrightarrow{\text{NaNO}2/\text{HCl}} \text{Diazonium salt} \xrightarrow{\text{NH}3} \text{3,5-(NO}2\text{)}2\text{C}6\text{H}3\text{NH}_2
Key advantages of this method include:
-
Reduced isomer formation : The chloro group directs nitration to the 3,5 positions before displacement .
-
Higher purity : Diazonium intermediates are isolated and purified, minimizing byproducts .
However, the requirement for anhydrous NH₃ and low-temperature conditions (−10°C to 5°C) complicates scalability .
Patent-Based Synthesis Using Sodium Azide
Source discloses a patent method involving sodium azide (NaN₃) and chloroform (CHCl₃) in oleum (fuming H₂SO₄). This approach converts 3,5-dinitrobenzoic acid to this compound via an intermediate acyl azide:
2\text{)}2\text{C}6\text{H}3\text{COOH} \xrightarrow{\text{NaN}3/\text{CHCl}3} \text{Acyl azide} \xrightarrow{\Delta} \text{3,5-(NO}2\text{)}2\text{C}6\text{H}3\text{NH}_2
Reaction Conditions :
-
Oleum concentration : 20% free SO₃ ensures sulfonation of the carboxylic acid, facilitating azide formation .
-
Temperature : 45–50°C during NaN₃ addition prevents premature decomposition of the azide intermediate .
This method achieves yields of 70–75% but requires careful handling of toxic HN₃ gas evolved during the reaction .
Solvent-Free Mechanochemical Synthesis
Recent advances explore solvent-free nitration using ball milling. A mixture of aniline, KNO₃, and clay catalysts (e.g., montmorillonite K10) is ground at 30 Hz for 2 hours, achieving 65% conversion to this compound . Advantages include:
-
Reduced waste : Eliminates acidic wastewater from traditional nitration .
-
Faster reaction times : 2 hours vs. 8–12 hours in solution-phase methods .
Purification and Characterization
Crude this compound is purified via recrystallization from ethanol/water (1:3 v/v), yielding yellow needles with a melting point of 160–163°C . Spectroscopic data confirm structure:
-
IR (KBr) : ν = 1536 cm⁻¹ (asymmetric NO₂ stretch), 1348 cm⁻¹ (symmetric NO₂ stretch) .
-
¹H NMR (DMSO-d₆) : δ 8.60 (s, 2H, Ar-H), 6.20 (s, 1H, NH₂) .
Table 2: Physical Properties of this compound
Análisis De Reacciones Químicas
Types of Reactions:
Reduction: 3,5-Dinitroaniline can undergo reduction reactions to form 3,5-diaminoaniline. Common reducing agents for this reaction include hydrogen gas in the presence of a catalyst such as palladium on carbon, or chemical reducing agents like iron and hydrochloric acid.
Substitution: The amino group in this compound can participate in nucleophilic substitution reactions. For example, it can react with acyl chlorides to form amides.
Oxidation: Although less common, this compound can be oxidized under strong conditions to form corresponding nitro compounds with higher oxidation states.
Common Reagents and Conditions:
Reduction: Hydrogen gas with palladium on carbon, iron with hydrochloric acid.
Substitution: Acyl chlorides, acid anhydrides.
Oxidation: Strong oxidizing agents like potassium permanganate.
Major Products:
Reduction: 3,5-Diaminoaniline.
Substitution: Amides and other substituted derivatives.
Oxidation: Higher oxidation state nitro compounds.
Aplicaciones Científicas De Investigación
Applications in Agriculture
2.1 Veterinary Medicine
One notable application of 3,5-dinitroaniline derivatives is in veterinary medicine for the prevention of coccidiosis in livestock. Research has shown that certain derivatives exhibit efficacy against coccidia, which are parasites that can cause significant health issues in animals .
2.2 Herbicide Development
This compound has been explored as a potential herbicide due to its ability to inhibit specific biochemical pathways in plants. Its derivatives can be modified to enhance selectivity and efficacy against target weed species while minimizing harm to crops .
Environmental and Toxicological Studies
3.1 Genotoxicity Testing
Studies have indicated that this compound exhibits mutagenic properties when tested on various strains of Salmonella typhimurium. It was found to induce mutations at concentrations ranging from 0.5 to 40 μg/plate, highlighting its potential risks as an environmental contaminant .
3.2 Toxicity Assessment
The U.S. Environmental Protection Agency (EPA) has conducted assessments on the toxicity of this compound, noting limited data on its effects in humans or animals. However, it has been classified as potentially hazardous based on its genotoxicity profile .
Study on Microbial Interactions
Research involving Azotobacter vinelandii demonstrated that this bacterium could metabolize munitions compounds like this compound, suggesting potential applications in bioremediation efforts to clean up contaminated environments .
Synthesis of Derivatives
A study detailed the synthesis of various derivatives from this compound using different reagents and conditions. The resulting compounds were evaluated for their biological activities, indicating that modifications could enhance their agricultural applications .
Data Tables
Mecanismo De Acción
Comparación Con Compuestos Similares
Physical Properties :
- Melting Point: 161°C .
- Purity Grades: Commercial grades (95–98%) are cost-effective for agrochemicals, while higher purity (>99%) is used in pharmaceuticals and dyes .
Structural and Functional Analogues
Table 1: Structural Comparison
Reactivity and Toxicity
Table 2: Toxicity and Environmental Impact
Market and Regulatory Trends
Table 3: Industrial Demand and Regulations
Key Research Findings
- Herbicide Resistance : Mutations in plant tubulin (e.g., Chlamydomonas reinhardtii) reduce this compound herbicide efficacy, necessitating higher doses .
- Pharmaceutical Derivatives : 3',5'-Dinitro-N-methylacetanilide (m.p. 134.5–136°C) shows superior stability in poultry feed compared to analogues .
- Environmental Detection : this compound is quantified at 576–1005 ppb in contaminated water using HPLC .
Actividad Biológica
3,5-Dinitroaniline (CASRN 618-87-1) is a chemical compound with significant biological activity, particularly in the context of its use as a herbicide and its potential toxicity to various organisms. This article provides a detailed examination of its biological effects, including genotoxicity, herbicidal properties, and interactions with microbial species.
Chemical Structure and Properties
This compound is characterized by two nitro groups attached to an aniline structure. This configuration contributes to its biological activity, particularly in inhibiting cellular processes in various organisms.
Genotoxicity Studies
Genotoxicity refers to the ability of a compound to damage genetic information within a cell. Studies have demonstrated that this compound exhibits mutagenic properties when tested on various strains of Salmonella typhimurium.
Key Findings:
- Mutagenicity : Positive results were observed in strains TA98 and TA100 at concentrations ranging from 0.5 to 40 μg/plate without metabolic activation. The compound induced a doubling of the spontaneous mutation rate at concentrations ≥34 μg/plate in TA98 and ≥17 μg/plate in TA100 .
- In Vitro Studies : A comprehensive study indicated that this compound was mutagenic across multiple strains, including TA1535, TA1537, and TA1538, suggesting a broad potential for genetic damage .
Test System | Concentrations Tested (μg/plate) | Results Without Activation | Results With Activation |
---|---|---|---|
Salmonella typhimurium TA98 | 0.5 - 40 | Positive | Positive |
Salmonella typhimurium TA100 | 0.5 - 40 | Positive | Positive |
Salmonella typhimurium TA1535 | 0.5 - 40 | Positive | Negative |
Herbicidal Activity
This compound is part of a class of herbicides known for their efficacy against various weeds and plants. The mechanism primarily involves disrupting microtubule formation during cell division.
Herbicidal Mechanism:
- Microtubule Disruption : The compound inhibits tubulin polymerization, which is essential for mitosis in plants. This action leads to stunted growth and eventual plant death .
- Selectivity : While effective against many weed species, research indicates that modifications to the dinitroaniline structure can enhance selectivity toward specific plant types while minimizing harm to crops .
Toxicological Profile
The toxicity of this compound varies among different species. Acute toxicity studies have shown that it can affect both terrestrial and aquatic organisms.
Toxicity Data:
- Terrestrial Species : Varying levels of toxicity have been reported in earthworms and insects. For example, exposure to certain concentrations resulted in significant bioaccumulation within tissues .
- Aquatic Species : In aquatic environments, compounds like pendimethalin (related to dinitroanilines) have shown rapid accumulation in gills and other tissues following exposure .
Microbial Interactions
Research has also explored the interactions between this compound and microbial species such as Azotobacter vinelandii. These interactions can influence nitrogen fixation processes and overall soil health.
Microbial Effects:
- Studies indicate that certain microbial strains can metabolize dinitroanilines, potentially reducing their environmental impact .
- The impact on microbial communities can alter soil nutrient dynamics and affect plant growth indirectly through changes in microbial activity.
Case Studies
Several case studies highlight the implications of using this compound in agricultural settings:
- Field Trials : Field studies assessing the efficacy of dinitroaniline-based herbicides showed significant reductions in weed populations while monitoring for non-target effects on crops.
- Ecotoxicological Assessments : Ecotoxicological evaluations revealed varying impacts on non-target species across different ecosystems, emphasizing the need for careful application practices.
Q & A
Basic Research Questions
Q. What are the validated analytical methods for detecting 3,5-dinitroaniline in environmental water samples?
A solid-phase extraction (SPE) coupled with high-performance liquid chromatography (HPLC) method has been optimized for detecting this compound in surface and drinking water. Key parameters include:
- Linear range : 1.0–50.0 mg/L.
- Detection limit : 0.5 µg/L.
- Recovery rates : 72.2–96.1% (dependent on mobile phase ratios and gradient elution protocols). Method validation requires optimization of chromatographic columns (e.g., C18 reverse-phase) and mobile phase composition (e.g., acetonitrile/water gradients) to resolve co-eluting nitroaromatic compounds .
Q. How can synthesis purity of this compound be ensured during ammonolysis reactions?
Impurities in synthetic routes (e.g., via ammonolysis of potassium 4-chloro-3,5-dinitrobenzenesulfonate) often arise from inadequate recrystallization. Best practices include:
- Pre-recrystallization of intermediates to remove sulfonate byproducts.
- Maintaining heated filtration systems (>60°C) to prevent premature crystallization.
- Post-synthesis purification via ethanol recrystallization (solubility: ~0.4 g/100 mL in 95% ethanol at 25°C) .
Q. What are the critical physical-chemical properties of this compound for experimental design?
Key properties include:
- Molecular weight : 183.12 g/mol.
- Melting point : 160–162°C.
- Solubility : Low in water (<0.1 g/L), moderate in polar organic solvents (e.g., acetonitrile, ethanol).
- Stability : Decomposes at >194°C; sensitive to photodegradation in aqueous environments. These properties inform solvent selection, storage conditions (10°C or colder for standards), and reaction temperature limits .
Advanced Research Questions
Q. How can discrepancies in environmental concentration measurements of this compound be resolved?
Variations in reported concentrations (e.g., 567–940 ppb in groundwater samples) may arise from:
- Matrix effects : Co-eluting contaminants (e.g., nitrobenzene, dinitrotoluene) in complex environmental samples.
- Instrument calibration : Use of certified reference standards (e.g., 1000 µg/mL in acetonitrile) and internal standards (e.g., deuterated analogs) to improve accuracy.
- Sample preparation : SPE recovery rates vary with pH and ionic strength; acidification to pH 2–3 enhances analyte retention .
Q. What are the photodegradation pathways of this compound in aqueous systems?
Under UV irradiation, this compound undergoes:
- Nitro group reduction : Formation of amino-nitro intermediates (e.g., 3-amino-5-nitroaniline).
- Ring cleavage : Production of low-molecular-weight carboxylic acids (e.g., oxalic acid).
- Polymerization : Formation of dimeric species via radical coupling. Advanced characterization techniques (e.g., 13C/15N NMR, LC-MS/MS) are required to track transformation products .
Q. How do computational models align with experimental data for nonlinear optical (NLO) properties of this compound?
Polarizable Continuum Model (PCM) simulations predict macroscopic susceptibilities by integrating molecular hyperpolarizabilities (β, γ) with solvent dielectric effects. For this compound:
- EFISH experiments : Third-order susceptibility (χ⁽³⁾) correlates with computed dipole moments.
- Discrepancies : Arise from solvent-molecule interactions (e.g., hydrogen bonding in polar solvents like water) not fully captured in ab initio models. Hybrid QM/MM approaches improve accuracy for NLO applications .
Q. What genetic engineering strategies target dinitroaniline herbicide resistance in crops?
Adenine base editors (ABEs) like TadA8e enable precise A→G mutations in α-tubulin genes, conferring resistance to dinitroaniline herbicides. Key steps include:
- Target selection : Editing conserved residues in tubulin binding pockets (e.g., Thr-349 in wheat).
- Efficiency optimization : Codon usage adaptation for polyploid species (e.g., allohexaploid wheat).
- Phenotypic validation : Root growth assays under herbicide stress (e.g., trifluralin exposure) .
Q. Methodological Notes
- Synthesis : Prioritize intermediates with electron-withdrawing groups (e.g., sulfonates) to enhance ammonolysis yields .
- Environmental Analysis : Use tandem mass spectrometry (MS/MS) to differentiate this compound from structural isomers (e.g., 2,4-dinitroaniline) .
- Computational Studies : Benchmark solvent effects using explicit solvent models (e.g., COSMO-RS) for NLO property predictions .
Propiedades
IUPAC Name |
3,5-dinitroaniline | |
---|---|---|
Source | PubChem | |
URL | https://pubchem.ncbi.nlm.nih.gov | |
Description | Data deposited in or computed by PubChem | |
InChI |
InChI=1S/C6H5N3O4/c7-4-1-5(8(10)11)3-6(2-4)9(12)13/h1-3H,7H2 | |
Source | PubChem | |
URL | https://pubchem.ncbi.nlm.nih.gov | |
Description | Data deposited in or computed by PubChem | |
InChI Key |
MPBZUKLDHPOCLS-UHFFFAOYSA-N | |
Source | PubChem | |
URL | https://pubchem.ncbi.nlm.nih.gov | |
Description | Data deposited in or computed by PubChem | |
Canonical SMILES |
C1=C(C=C(C=C1[N+](=O)[O-])[N+](=O)[O-])N | |
Source | PubChem | |
URL | https://pubchem.ncbi.nlm.nih.gov | |
Description | Data deposited in or computed by PubChem | |
Molecular Formula |
C6H5N3O4 | |
Source | PubChem | |
URL | https://pubchem.ncbi.nlm.nih.gov | |
Description | Data deposited in or computed by PubChem | |
DSSTOX Substance ID |
DTXSID0044151 | |
Record name | 3,5-Dinitroaniline | |
Source | EPA DSSTox | |
URL | https://comptox.epa.gov/dashboard/DTXSID0044151 | |
Description | DSSTox provides a high quality public chemistry resource for supporting improved predictive toxicology. | |
Molecular Weight |
183.12 g/mol | |
Source | PubChem | |
URL | https://pubchem.ncbi.nlm.nih.gov | |
Description | Data deposited in or computed by PubChem | |
CAS No. |
618-87-1 | |
Record name | 3,5-Dinitroaniline | |
Source | CAS Common Chemistry | |
URL | https://commonchemistry.cas.org/detail?cas_rn=618-87-1 | |
Description | CAS Common Chemistry is an open community resource for accessing chemical information. Nearly 500,000 chemical substances from CAS REGISTRY cover areas of community interest, including common and frequently regulated chemicals, and those relevant to high school and undergraduate chemistry classes. This chemical information, curated by our expert scientists, is provided in alignment with our mission as a division of the American Chemical Society. | |
Explanation | The data from CAS Common Chemistry is provided under a CC-BY-NC 4.0 license, unless otherwise stated. | |
Record name | 3,5-Dinitroaniline | |
Source | ChemIDplus | |
URL | https://pubchem.ncbi.nlm.nih.gov/substance/?source=chemidplus&sourceid=0000618871 | |
Description | ChemIDplus is a free, web search system that provides access to the structure and nomenclature authority files used for the identification of chemical substances cited in National Library of Medicine (NLM) databases, including the TOXNET system. | |
Record name | 3,5-DINITROANILINE | |
Source | DTP/NCI | |
URL | https://dtp.cancer.gov/dtpstandard/servlet/dwindex?searchtype=NSC&outputformat=html&searchlist=284 | |
Description | The NCI Development Therapeutics Program (DTP) provides services and resources to the academic and private-sector research communities worldwide to facilitate the discovery and development of new cancer therapeutic agents. | |
Explanation | Unless otherwise indicated, all text within NCI products is free of copyright and may be reused without our permission. Credit the National Cancer Institute as the source. | |
Record name | 3,5-Dinitroaniline | |
Source | EPA DSSTox | |
URL | https://comptox.epa.gov/dashboard/DTXSID0044151 | |
Description | DSSTox provides a high quality public chemistry resource for supporting improved predictive toxicology. | |
Record name | 3,5-dinitroaniline | |
Source | European Chemicals Agency (ECHA) | |
URL | https://echa.europa.eu/substance-information/-/substanceinfo/100.009.608 | |
Description | The European Chemicals Agency (ECHA) is an agency of the European Union which is the driving force among regulatory authorities in implementing the EU's groundbreaking chemicals legislation for the benefit of human health and the environment as well as for innovation and competitiveness. | |
Explanation | Use of the information, documents and data from the ECHA website is subject to the terms and conditions of this Legal Notice, and subject to other binding limitations provided for under applicable law, the information, documents and data made available on the ECHA website may be reproduced, distributed and/or used, totally or in part, for non-commercial purposes provided that ECHA is acknowledged as the source: "Source: European Chemicals Agency, http://echa.europa.eu/". Such acknowledgement must be included in each copy of the material. ECHA permits and encourages organisations and individuals to create links to the ECHA website under the following cumulative conditions: Links can only be made to webpages that provide a link to the Legal Notice page. | |
Record name | 3,5-DINITROANILINE | |
Source | FDA Global Substance Registration System (GSRS) | |
URL | https://gsrs.ncats.nih.gov/ginas/app/beta/substances/0AHR6K1N73 | |
Description | The FDA Global Substance Registration System (GSRS) enables the efficient and accurate exchange of information on what substances are in regulated products. Instead of relying on names, which vary across regulatory domains, countries, and regions, the GSRS knowledge base makes it possible for substances to be defined by standardized, scientific descriptions. | |
Explanation | Unless otherwise noted, the contents of the FDA website (www.fda.gov), both text and graphics, are not copyrighted. They are in the public domain and may be republished, reprinted and otherwise used freely by anyone without the need to obtain permission from FDA. Credit to the U.S. Food and Drug Administration as the source is appreciated but not required. | |
Synthesis routes and methods I
Procedure details
Synthesis routes and methods II
Procedure details
Synthesis routes and methods III
Procedure details
Synthesis routes and methods IV
Procedure details
Retrosynthesis Analysis
AI-Powered Synthesis Planning: Our tool employs the Template_relevance Pistachio, Template_relevance Bkms_metabolic, Template_relevance Pistachio_ringbreaker, Template_relevance Reaxys, Template_relevance Reaxys_biocatalysis model, leveraging a vast database of chemical reactions to predict feasible synthetic routes.
One-Step Synthesis Focus: Specifically designed for one-step synthesis, it provides concise and direct routes for your target compounds, streamlining the synthesis process.
Accurate Predictions: Utilizing the extensive PISTACHIO, BKMS_METABOLIC, PISTACHIO_RINGBREAKER, REAXYS, REAXYS_BIOCATALYSIS database, our tool offers high-accuracy predictions, reflecting the latest in chemical research and data.
Strategy Settings
Precursor scoring | Relevance Heuristic |
---|---|
Min. plausibility | 0.01 |
Model | Template_relevance |
Template Set | Pistachio/Bkms_metabolic/Pistachio_ringbreaker/Reaxys/Reaxys_biocatalysis |
Top-N result to add to graph | 6 |
Feasible Synthetic Routes
Descargo de responsabilidad e información sobre productos de investigación in vitro
Tenga en cuenta que todos los artículos e información de productos presentados en BenchChem están destinados únicamente con fines informativos. Los productos disponibles para la compra en BenchChem están diseñados específicamente para estudios in vitro, que se realizan fuera de organismos vivos. Los estudios in vitro, derivados del término latino "in vidrio", involucran experimentos realizados en entornos de laboratorio controlados utilizando células o tejidos. Es importante tener en cuenta que estos productos no se clasifican como medicamentos y no han recibido la aprobación de la FDA para la prevención, tratamiento o cura de ninguna condición médica, dolencia o enfermedad. Debemos enfatizar que cualquier forma de introducción corporal de estos productos en humanos o animales está estrictamente prohibida por ley. Es esencial adherirse a estas pautas para garantizar el cumplimiento de los estándares legales y éticos en la investigación y experimentación.