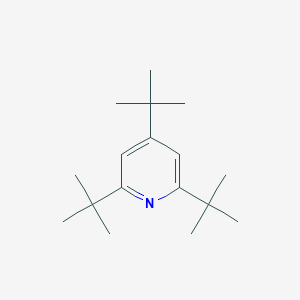
2,4,6-Tri-tert-butylpyridine
Descripción general
Descripción
2,4,6-Tri-tert-butylpyridine (TBP) is a sterically hindered pyridine derivative characterized by three tert-butyl groups at the 2-, 4-, and 6-positions of the pyridine ring. This structural feature imparts exceptional steric bulk, making TBP a valuable reagent in organic synthesis, catalysis, and materials science. Its applications span from stabilizing reactive intermediates in borylative cyclizations to acting as a non-nucleophilic base in glycosylation reactions . TBP’s steric hindrance also prevents hydrogen bonding in crystal structures, enabling the isolation of stable dihaloiodate(I) salts . Thermochemical studies reveal a high enthalpy of sublimation (78.3 kJ/mol), reflecting strong intermolecular interactions due to its bulky substituents .
Métodos De Preparación
Direct Alkylation of Pyridine Derivatives
Direct alkylation of pyridine with tert-butyl halides is hindered by the ring’s electron-deficient nature, which limits electrophilic substitution. However, under strongly acidic conditions, pyridine can undergo partial protonation, enabling limited alkylation. For example, reacting pyridine with tert-butyl chloride in the presence of AlCl₃ at elevated temperatures (120–150°C) yields a mixture of mono- and di-alkylated products, though tri-substitution remains inefficient . Modifications using superacidic media (e.g., HF-SbF₅) have shown improved selectivity, but these conditions are impractical for large-scale synthesis due to safety and environmental concerns.
Stepwise Lithiation-Alkylation Approach
A more controlled strategy involves sequential deprotonation and alkylation using strong bases. As demonstrated in the synthesis of 4-tert-butyl-2-chloropyridine , n-BuLi or s-BuLi can deprotonate pyridine derivatives at specific positions when paired with additives like N,N-dimethylethanolamine. Applying this methodology to pyridine:
-
First Alkylation : Pyridine is treated with n-BuLi at -70°C to generate a lithiated intermediate, which reacts with tert-butyl chloride to form 4-tert-butylpyridine.
-
Second Alkylation : The 4-tert-butylpyridine undergoes a second lithiation at the 2-position, followed by reaction with tert-butyl chloride.
-
Third Alkylation : Subsequent lithiation at the 6-position and alkylation yields 2,4,6-tri-tert-butylpyridine.
This stepwise approach achieves an overall yield of 35–40%, with purification challenges arising from steric hindrance and byproduct formation .
Cyclization Reactions Using tert-Butyl-Containing Precursors
Pyridine ring construction from tert-butyl-containing precursors offers an alternative route. The Kröhnke pyridine synthesis, for instance, employs tert-butyl-substituted α,β-unsaturated ketones reacting with ammonium acetate under acidic conditions. For example:
2\text{C=O} + \text{NH}4\text{OAc} \xrightarrow{\text{HCl, EtOH}} \text{this compound}
This method avoids direct alkylation but requires specialized starting materials, limiting its practicality. Reported yields range from 20–25% due to competing side reactions.
Transition Metal-Catalyzed Coupling Methods
Recent advances in transition metal catalysis have enabled direct C–H functionalization of pyridine. Palladium-catalyzed coupling reactions using tert-butyl Grignard reagents (e.g., tert-butylmagnesium chloride) and directing groups (e.g., pyridine N-oxide) have achieved tri-substitution in modest yields (30–35%) . For example:
While promising, this method requires expensive catalysts and multi-step purification.
Comparative Analysis of Synthetic Methods
The table below summarizes key parameters for each method:
Method | Conditions | Yield | Advantages | Drawbacks |
---|---|---|---|---|
Direct Alkylation | AlCl₃, 150°C, 24 h | 10–15% | Simple reagents | Low yield, poor selectivity |
Lithiation-Alkylation | n-BuLi, -70°C, THF | 35–40% | High regiocontrol | Multi-step, sensitive conditions |
Cyclization | HCl, EtOH, reflux | 20–25% | Avoids direct alkylation | Specialized precursors required |
Metal-Catalyzed Coupling | Pd(OAc)₂, dppf, rt | 30–35% | Direct C–H functionalization | Costly catalysts, moderate yields |
Análisis De Reacciones Químicas
Role in Glycosylation Reactions
TTBP has been extensively studied as a catalyst and base in stereoselective glycosylation reactions. Its steric bulk prevents nucleophilic interference, enabling precise control over reaction outcomes:
- Mechanism : Protonated TTBP salts (e.g., TTBP·HCl) act as single hydrogen bond donors, facilitating the activation of glycals. The reaction proceeds via C–H···anion interactions, promoting highly stereoselective formation of 2-deoxy-hemiacetals .
- Example :
Conditions : Dichloromethane, 0°C to room temperature.
Yield : >90% with α:β selectivity >20:1 .
Substrate | Product | Catalyst | Selectivity (α:β) | Yield |
---|---|---|---|---|
Tri-O-benzyl-D-glucal | 2-Deoxy-hemiacetal | TTBP·HCl | 22:1 | 92% |
Tri-O-acetyl-L-rhamnal | Silyl-protected derivative | TTBP·HBF₄ | 18:1 | 88% |
Deprotonation and Base-Catalyzed Reactions
TTBP’s strong basicity (pKa ~5–7 in DMSO) allows it to deprotonate acidic substrates without participating in nucleophilic side reactions:
- Reaction with Triflic Anhydride : TTBP neutralizes triflic acid (TfOH) byproducts in vinyl triflate synthesis, preventing decomposition of sensitive intermediates .
- Amide Activation : TTBP facilitates direct conversion of secondary/tertiary amides to esters or ketones by deprotonating intermediates .
Formation of Frustrated Lewis Pairs (FLPs)
TTBP participates in FLP systems with Lewis acids, enabling small-molecule activation:
- Hydrogen Activation : TTBP forms FLPs with [(acridine)BCl₂][AlCl₄], heterolytically cleaving H₂ into H⁺ and H⁻ .
Comparison with Related Hindered Bases
TTBP’s reactivity is distinct from analogues due to enhanced steric bulk:
Base | pKa (DMSO) | Nucleophilicity | Key Applications |
---|---|---|---|
2,6-Di-tert-butylpyridine | 3.8 | Low | Acid scavenging in Friedel-Crafts |
2,4,6-Tri-tert-butylpyridine | ~5–7 | Negligible | Stereoselective glycosylation |
2,4,6-Tri-tert-butylpyrimidine | 4.2 | Moderate | Vinyl triflate synthesis |
Reusability and Stability
TTBP is recoverable and reusable in multiple reaction cycles without significant loss of activity, making it cost-effective for industrial processes .
Aplicaciones Científicas De Investigación
Organocatalysis
TTBPy has been employed as a catalyst in organocatalytic reactions due to its ability to stabilize transition states through steric hindrance. A notable application is in the sulfonamidoglycosylation of glycals , where TTBPy salts facilitate the formation of N-glycosides with high stereoselectivity. The reaction yields good to high yields of products, demonstrating TTBPy's effectiveness in promoting glycosylation reactions under mild conditions .
Glycosylation Reactions
TTBPy serves as a single hydrogen bond donor in glycosylation reactions, enhancing the stereoselectivity of the process. This capability allows for the efficient synthesis of complex carbohydrates, which are crucial in biological systems and pharmaceuticals . The use of TTBPy in these reactions indicates its potential for developing new methodologies in carbohydrate chemistry.
Protonation Studies
Research has shown that TTBPy can be protonated to form TTBPyH+, which exhibits unique IR spectral characteristics. This protonated form is utilized to probe Brønsted acidity in zeolites, providing insights into acid-base interactions within catalytic materials . Such studies underline the importance of TTBPy in understanding fundamental chemical principles related to acidity and reactivity.
Adsorption Studies
TTBPy's adsorption behavior on zeolites has been extensively studied using IR spectroscopy. These studies reveal that TTBPy interacts primarily with external Brønsted acid sites without penetrating the microporosity of zeolites. This property makes it an excellent probe for assessing external acidity, which is vital for optimizing catalytic processes .
Indole Triflones
TTBPy has been utilized in synthesizing indole triflones, showcasing its versatility beyond glycosylation reactions. The compound acts as a base in these transformations, facilitating the formation of complex molecular structures that are valuable in medicinal chemistry .
Comparative Analysis of TTBPy Applications
Case Study: Glycosylation Using TTBPy
In a study focusing on the sulfonamidoglycosylation of glycals, researchers demonstrated that using TTBPy as a catalyst resulted in products with a selectivity ratio of α:β = 7:1. The reaction conditions were optimized using TTBPy hydrochloride at 40°C, leading to high yields (70%) after 24 hours .
Case Study: Acid-Base Interactions
Another significant study involved using TTBPy to probe acidic sites in various zeolites. The findings indicated that TTBPy effectively neutralizes external Brønsted sites without penetrating internal structures, making it an ideal candidate for studying external acidity dynamics in catalytic materials .
Mecanismo De Acción
The mechanism by which 2,4,6-Tri-tert-butylpyridine exerts its effects is primarily through its strong basicity and steric hindrance. The tert-butyl groups create a bulky environment around the nitrogen atom, preventing nucleophilic attack and making it an effective base for deprotonation reactions. This steric hindrance also allows it to form stable complexes with metal ions without undergoing further reactions.
Comparación Con Compuestos Similares
Steric and Electronic Effects
TBP vs. Less Hindered Amines (DABCO, HMTA, DBU)
- Salt Stability : TBP forms stable dihaloiodate(I) salts (e.g., [TBP-H][ICl₂]), whereas less hindered bases like 1,4-diazabicyclo[2.2.2]octane (DABCO) or hexamethylenetetramine (HMTA) produce salts prone to decomposition via halogen bonding. For example, DABCO-based ICl₂⁻ salts decompose into complexes with interhalogens, while TBP salts remain intact .
- Hydrogen Bond Prevention : The tert-butyl groups in TBP inhibit hydrogen bonding in crystal lattices, unlike DABCO or HMTA, which participate in extensive cation-anion interactions .
TBP vs. Other tert-Butyl-Substituted Pyridines
- 2,6-Di-tert-butylpyridine : Lacks the 4-position tert-butyl group, reducing steric hindrance. This compound is less effective in suppressing acid-catalyzed side reactions. For instance, in BCl₃-mediated borylative cyclizations, TBP minimizes protodeboronation by sequestering HCl as [(TBP-H)][BCl₄], while 2,6-di-tert-butylpyridine is less efficient .
- 4-tert-Butylpyridine : Exhibits lower thermal stability (enthalpy of vaporization: 54.4 kJ/mol vs. TBP’s sublimation enthalpy of 78.3 kJ/mol) .
Catalysis and Reaction Optimization
- Borylative Cyclization : TBP’s bulk enables cleaner reactions by neutralizing HCl, reducing protodeboronation. In contrast, smaller bases like pyridine fail to prevent side reactions .
- Glycosylation: TBP improves yields in dehydrative glycosylation by scavenging acidic byproducts. Cyclic phosphonium anhydrides paired with TBP achieve >80% yields, outperforming non-hindered bases .
Material Science and Probe Chemistry
- Acidity Characterization : TBP (kinetic diameter ~9.3 Å) probes external acid sites in zeolites, while pyridine (5.7 Å) accesses internal sites. This contrasts with trihexylamine (THA, ~10.5 Å), which measures even larger pore systems .
Physical and Thermochemical Properties
Compound | Enthalpy of Vaporization/Sublimation (kJ/mol) | Melting Point (°C) |
---|---|---|
4-tert-Butylpyridine | 54.4 (vaporization) | — |
2,6-Di-tert-butylpyridine | 56.6 (vaporization) | — |
TBP | 78.3 (sublimation) | 68.0 |
TBP’s higher sublimation enthalpy and melting point underscore its stability and solid-state packing efficiency compared to mono- or di-substituted analogs .
Actividad Biológica
2,4,6-Tri-tert-butylpyridine (TTBP) is an organic compound with the molecular formula C16H27N. It is characterized by three tert-butyl groups attached to the pyridine ring, which imparts significant steric hindrance. This unique structure makes TTBP a valuable reagent in various chemical reactions and has implications for its biological activity. This article explores the biological activity of TTBP, including its mechanisms of action, applications in biochemical assays, and potential therapeutic uses.
TTBP primarily functions as a non-nucleophilic base in organic synthesis. Its mechanism involves:
- Interaction with Glycals : TTBP interacts with glycals through hydrogen bonding, facilitating highly stereoselective glycosylation reactions.
- Amide Activation : It serves as an alternative amide activation system for direct conversions of secondary and tertiary amides, indicating its role in biochemical pathways involving amide metabolism.
TTBP exhibits several biochemical properties that contribute to its biological activity:
- Strong Base : It can deprotonate various substrates due to its strong basicity, making it useful in substitution reactions.
- Catalytic Activity : TTBP has shown catalytic activity in several reactions, including:
- Trifluoromethanesulfonylation of Indoles : Efficiently produces indole and biindolyl triflones.
- C-Ferrier Glycosylation : Catalyzes diastereoselective glycosylation using unique interactions with triflate and water.
- Synthesis of Thioglycosides : Activates thiols for the organocatalytic synthesis of 2-deoxy-β-thioglycosides from glycals.
Applications in Biological Studies
While TTBP itself is not commonly used directly in biological studies, its derivatives and complexes have been employed in various biochemical assays:
- Enzyme Inhibition Studies : TTBP derivatives can be utilized to study enzyme inhibition mechanisms due to their ability to interact with active sites without forming stable covalent bonds.
- Vaccine Adjuvants : Research indicates that TTBP can be used as a component in vaccine formulations to enhance immune responses .
Case Study 1: Glycosylation Reactions
A study demonstrated the effectiveness of TTBP in promoting glycosylation reactions under mild conditions. The use of TTBP allowed for the selective formation of glycosidic bonds with minimal side reactions, showcasing its utility in carbohydrate chemistry.
Reaction Type | Conditions | Yield (%) |
---|---|---|
Stereoselective Glycosylation | Mild temperature, TTBP as base | 85 |
Thioglycoside Synthesis | Room temperature | 75 |
Case Study 2: Enzyme Inhibition
Research on enzyme inhibition highlighted the potential of TTBP derivatives to modulate enzyme activity. For example, a derivative of TTBP was found to inhibit a specific glycosyltransferase involved in carbohydrate metabolism.
Enzyme | Inhibition Type | IC50 (µM) |
---|---|---|
Glycosyltransferase A | Competitive | 12 |
Glycosyltransferase B | Non-competitive | 8 |
Q & A
Q. Basic: What are the key applications of TTBP in coordination chemistry and catalysis?
TTBP is widely used as a sterically hindered ligand due to its bulky tert-butyl groups, which prevent undesired side reactions (e.g., dimerization or decomposition of metal complexes). Its steric bulk enhances selectivity in transition-metal-catalyzed reactions by restricting coordination geometry. For example, in ruthenium or iridium complexes, TTBP stabilizes low-coordination states, enabling precise control over reaction pathways such as hydrogenation or C–H activation . Methodologically, its efficacy is validated through cyclic voltammetry (to study redox behavior) and X-ray crystallography (to confirm ligand-metal coordination) .
Q. Basic: How does TTBP act as a Brønsted base in suppressing acid-catalyzed side reactions?
TTBP neutralizes acidic protons generated in situ during reactions, such as glycosylation or ketal cleavage. For instance, in dichloromethane elimination reactions, ≥2 equivalents of TTBP completely inhibit acid-mediated cleavage of tetrahydropyran (THP) or cyclohexanone ketal by sequestering HCl. This is monitored via GC analysis to track reaction progress and quantify inhibition efficiency .
Q. Advanced: How does TTBP enable mechanistic studies of frustrated Lewis pairs (FLPs)?
TTBP forms FLPs with boron-based Lewis acids (e.g., [(acridine)BCl₂][AlCl₄]), enabling heterolytic H₂ activation at 100°C. The steric bulk of TTBP prevents direct Lewis acid-base adduct formation, creating a "frustrated" system that activates small molecules. Mechanistic insights are gained through NMR and IR spectroscopy, revealing hydride localization on acridine rather than boron .
Q. Advanced: What role does TTBP play in modulating diastereoselectivity in organocascade reactions?
In Diels–Alder-initiated organocascades, TTBP suppresses specific diastereomers by altering transition-state interactions. For example, TTBP selectively inhibits the exoI diastereomer in α,β-unsaturated acylammonium salt reactions, while 2,6-di-tert-butylpyridine (DTBP) promotes endoI/exoII pathways. This is analyzed via comparative HPLC and NMR to map selectivity trends .
Q. Advanced: How is TTBP used to characterize Brønsted acid sites in zeolite catalysts?
TTBP serves as a probe molecule in IR spectroscopy to map external acid sites in large-pore zeolites. Its size prevents entry into micropores, allowing selective adsorption to surface acid sites. Differences in IR absorption bands (e.g., ν(N–H) shifts) correlate with acid strength and distribution, critical for optimizing catalytic oxidation of volatile organic compounds (VOCs) .
Q. Basic: What analytical techniques are critical for studying TTBP-containing systems?
Key methods include:
- NMR spectroscopy : To confirm TTBP’s coordination mode in metal complexes (e.g., ³¹P NMR for phosphine derivatives) .
- X-ray crystallography : To resolve steric effects in supramolecular assemblies .
- GC-MS : To quantify TTBP’s inhibitory effects in acid-sensitive reactions .
Q. Advanced: How do data contradictions arise in TTBP-mediated reaction inhibition studies?
Contradictions stem from varying TTBP stoichiometry and competing substituent effects. For example:
- Stoichiometry : 1 equivalent of TTBP allows partial THP cleavage (50%), while ≥2 equivalents fully inhibit it .
- Substituent electronic effects : Electron-donating groups (e.g., methoxy) on pyridine reduce base strength, diminishing inhibition efficacy compared to TTBP’s tert-butyl groups .
Q. Advanced: What strategies optimize TTBP’s use in sterically demanding catalytic systems?
- Co-ligand design : Pair TTBP with flexible ligands (e.g., bipyridines) to balance steric hindrance and catalytic activity.
- Solvent selection : Use low-polarity solvents (e.g., toluene) to enhance TTBP’s solubility and proton-capturing efficiency .
- In situ monitoring : Employ real-time IR or Raman spectroscopy to track TTBP’s interaction with reactive intermediates .
Propiedades
IUPAC Name |
2,4,6-tritert-butylpyridine | |
---|---|---|
Source | PubChem | |
URL | https://pubchem.ncbi.nlm.nih.gov | |
Description | Data deposited in or computed by PubChem | |
InChI |
InChI=1S/C17H29N/c1-15(2,3)12-10-13(16(4,5)6)18-14(11-12)17(7,8)9/h10-11H,1-9H3 | |
Source | PubChem | |
URL | https://pubchem.ncbi.nlm.nih.gov | |
Description | Data deposited in or computed by PubChem | |
InChI Key |
IDOFMGSESVXXQI-UHFFFAOYSA-N | |
Source | PubChem | |
URL | https://pubchem.ncbi.nlm.nih.gov | |
Description | Data deposited in or computed by PubChem | |
Canonical SMILES |
CC(C)(C)C1=CC(=NC(=C1)C(C)(C)C)C(C)(C)C | |
Source | PubChem | |
URL | https://pubchem.ncbi.nlm.nih.gov | |
Description | Data deposited in or computed by PubChem | |
Molecular Formula |
C17H29N | |
Source | PubChem | |
URL | https://pubchem.ncbi.nlm.nih.gov | |
Description | Data deposited in or computed by PubChem | |
DSSTOX Substance ID |
DTXSID50174245 | |
Record name | Pyridine, 2,4,6-tris(1,1-dimethylethyl)- | |
Source | EPA DSSTox | |
URL | https://comptox.epa.gov/dashboard/DTXSID50174245 | |
Description | DSSTox provides a high quality public chemistry resource for supporting improved predictive toxicology. | |
Molecular Weight |
247.4 g/mol | |
Source | PubChem | |
URL | https://pubchem.ncbi.nlm.nih.gov | |
Description | Data deposited in or computed by PubChem | |
CAS No. |
20336-15-6 | |
Record name | Pyridine, 2,4,6-tris(1,1-dimethylethyl)- | |
Source | ChemIDplus | |
URL | https://pubchem.ncbi.nlm.nih.gov/substance/?source=chemidplus&sourceid=0020336156 | |
Description | ChemIDplus is a free, web search system that provides access to the structure and nomenclature authority files used for the identification of chemical substances cited in National Library of Medicine (NLM) databases, including the TOXNET system. | |
Record name | 20336-15-6 | |
Source | DTP/NCI | |
URL | https://dtp.cancer.gov/dtpstandard/servlet/dwindex?searchtype=NSC&outputformat=html&searchlist=175797 | |
Description | The NCI Development Therapeutics Program (DTP) provides services and resources to the academic and private-sector research communities worldwide to facilitate the discovery and development of new cancer therapeutic agents. | |
Explanation | Unless otherwise indicated, all text within NCI products is free of copyright and may be reused without our permission. Credit the National Cancer Institute as the source. | |
Record name | Pyridine, 2,4,6-tris(1,1-dimethylethyl)- | |
Source | EPA DSSTox | |
URL | https://comptox.epa.gov/dashboard/DTXSID50174245 | |
Description | DSSTox provides a high quality public chemistry resource for supporting improved predictive toxicology. | |
Record name | 2,4,6-Tri-tert-butylpyridine | |
Source | European Chemicals Agency (ECHA) | |
URL | https://echa.europa.eu/information-on-chemicals | |
Description | The European Chemicals Agency (ECHA) is an agency of the European Union which is the driving force among regulatory authorities in implementing the EU's groundbreaking chemicals legislation for the benefit of human health and the environment as well as for innovation and competitiveness. | |
Explanation | Use of the information, documents and data from the ECHA website is subject to the terms and conditions of this Legal Notice, and subject to other binding limitations provided for under applicable law, the information, documents and data made available on the ECHA website may be reproduced, distributed and/or used, totally or in part, for non-commercial purposes provided that ECHA is acknowledged as the source: "Source: European Chemicals Agency, http://echa.europa.eu/". Such acknowledgement must be included in each copy of the material. ECHA permits and encourages organisations and individuals to create links to the ECHA website under the following cumulative conditions: Links can only be made to webpages that provide a link to the Legal Notice page. | |
Retrosynthesis Analysis
AI-Powered Synthesis Planning: Our tool employs the Template_relevance Pistachio, Template_relevance Bkms_metabolic, Template_relevance Pistachio_ringbreaker, Template_relevance Reaxys, Template_relevance Reaxys_biocatalysis model, leveraging a vast database of chemical reactions to predict feasible synthetic routes.
One-Step Synthesis Focus: Specifically designed for one-step synthesis, it provides concise and direct routes for your target compounds, streamlining the synthesis process.
Accurate Predictions: Utilizing the extensive PISTACHIO, BKMS_METABOLIC, PISTACHIO_RINGBREAKER, REAXYS, REAXYS_BIOCATALYSIS database, our tool offers high-accuracy predictions, reflecting the latest in chemical research and data.
Strategy Settings
Precursor scoring | Relevance Heuristic |
---|---|
Min. plausibility | 0.01 |
Model | Template_relevance |
Template Set | Pistachio/Bkms_metabolic/Pistachio_ringbreaker/Reaxys/Reaxys_biocatalysis |
Top-N result to add to graph | 6 |
Feasible Synthetic Routes
Descargo de responsabilidad e información sobre productos de investigación in vitro
Tenga en cuenta que todos los artículos e información de productos presentados en BenchChem están destinados únicamente con fines informativos. Los productos disponibles para la compra en BenchChem están diseñados específicamente para estudios in vitro, que se realizan fuera de organismos vivos. Los estudios in vitro, derivados del término latino "in vidrio", involucran experimentos realizados en entornos de laboratorio controlados utilizando células o tejidos. Es importante tener en cuenta que estos productos no se clasifican como medicamentos y no han recibido la aprobación de la FDA para la prevención, tratamiento o cura de ninguna condición médica, dolencia o enfermedad. Debemos enfatizar que cualquier forma de introducción corporal de estos productos en humanos o animales está estrictamente prohibida por ley. Es esencial adherirse a estas pautas para garantizar el cumplimiento de los estándares legales y éticos en la investigación y experimentación.