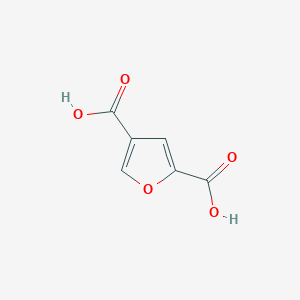
Ácido 2,4-furanodicarboxílico
Descripción general
Descripción
2,4-Furandicarboxylic acid (FDCA) is a compound with the molecular formula C6H4O5 . It has an average mass of 156.093 Da and a monoisotopic mass of 156.005875 Da . FDCA has received increasing attention as a significant platform chemical that can potentially replace petroleum-derived terephthalate acid (TPA) and produce green polymers such as polyethylene-2,5-furandicarboxylate (PEF) .
Synthesis Analysis
The synthesis of FDCA has been explored through various methods including chemocatalysis, biocatalysis, photocatalysis, and electrocatalysis . Biocatalysis is a promising approach with advantages that include mild reaction conditions, lower cost, higher selectivity, and environmental friendliness . The synthesis of FDCA starting from furoic acid and CO2 using different catalytic and stoichiometric processes has been reported .
Molecular Structure Analysis
The molecular structure of FDCA consists of a furan ring with carboxylic acid groups at the 2 and 4 positions . The exact structure can be represented by the InChI string: InChI=1S/C6H4O5/c7-5(8)3-1-4(6(9)10)11-2-3/h1-2H,(H,7,8)(H,9,10) .
Chemical Reactions Analysis
The chemical reactions involving FDCA have been studied extensively. For instance, the electrocatalytic synthesis of FDCA from 5-hydroxymethylfurfural (HMF) has been explored, which involves the oxidation of HMF under appropriate potential and ambient temperature . Other studies have focused on the conversion of furoic acid into FDCA through carboxylation reactions .
Physical And Chemical Properties Analysis
FDCA has a density of 1.6±0.1 g/cm3, a boiling point of 416.4±30.0 °C at 760 mmHg, and a vapour pressure of 0.0±1.0 mmHg at 25°C . It has a molar refractivity of 32.4±0.3 cm3, a polar surface area of 88 Å2, and a molar volume of 97.3±3.0 cm3 .
Aplicaciones Científicas De Investigación
Producción de bioplásticos
El FDCA es un componente clave en la producción de poliésteres de origen biológico como el poli(etilén furanoato) (PEF). El PEF se considera una alternativa más ecológica a los polímeros derivados del petróleo como el tereftalato de polietileno (PET), ofreciendo propiedades similares o superiores .
Marcos Metal-Orgánicos (MOFs)
El FDCA se ha utilizado en la síntesis de MOFs. Estos son materiales porosos con posibles aplicaciones en almacenamiento de gas, separación y catálisis .
Conversión de biomasa
Como un producto químico de plataforma en la conversión de biomasa y biorrefinerías, el FDCA juega un papel en la creación de soluciones sostenibles para los desafíos energéticos y ambientales .
Envasado de alimentos
Debido a sus propiedades de barrera, los polímeros derivados del FDCA como el PEF se están explorando para aplicaciones de contacto con alimentos para mejorar la seguridad alimentaria y prolongar la vida útil .
Poliésteres biodegradables
El FDCA contribuye al desarrollo de poliésteres biodegradables destinados a reducir la dependencia de los recursos fósiles y mejorar la sostenibilidad ambiental .
Copolymerización
La copolymerización del FDCA con otros monómeros puede ajustar las propiedades de los poliésteres para aplicaciones específicas .
Mecanismo De Acción
Target of Action
2,4-Furandicarboxylic acid (FDCA) is a bio-based platform chemical that has received increasing attention due to its potential to replace petroleum-derived terephthalate acid (TPA) in the production of green polymers . Its primary targets are the biochemical pathways involved in the synthesis of these polymers .
Mode of Action
The mode of action of 2,4-FDCA involves its interaction with various catalysts and reactants in the synthesis of bio-based polymers . For instance, it can be produced from the disproportionation of potassium-2-furoate at 260 °C in the presence of Lewis acidic catalysts like CdI2 or ZnCl2 . This reaction results in the formation of furan and furandicarboxylic acids .
Biochemical Pathways
The biochemical pathways affected by 2,4-FDCA primarily involve the synthesis of bio-based polymers . For instance, it can potentially replace petroleum-derived terephthalate acid (TPA) in the production of green polymers such as polyethylene-2,5-furandicarboxylate (PEF) . Furthermore, it has been suggested that 2,5-FDCA and 2,4-FDCA are formed by separate reaction pathways .
Pharmacokinetics
Its bioavailability and stability can be influenced by various factors, including the reaction conditions and the presence of catalysts .
Result of Action
The result of 2,4-FDCA’s action is the production of bio-based polymers, which can potentially replace petroleum-derived polymers . These green polymers, such as polyethylene-2,5-furandicarboxylate (PEF), have comparable properties to their petroleum-derived counterparts .
Action Environment
The action of 2,4-FDCA is influenced by various environmental factors, including the reaction conditions and the presence of catalysts . For instance, the selectivity towards the different FDCA isomers is affected by the type of catalyst used . Furthermore, the reaction conditions, such as temperature and the presence of other reactants, can also influence the yield and efficiency of 2,4-FDCA production .
Safety and Hazards
Direcciones Futuras
FDCA has been recognized as a promising bio-based building block for the production of sustainable polymers . Research is ongoing to develop more efficient biocatalytic production methods for FDCA . Furthermore, the synthesis of FDCA from renewable resources like lignocellulosic biomass is being explored to reduce dependency on fossil fuels .
Análisis Bioquímico
Biochemical Properties
2,4-Furandicarboxylic acid is involved in the synthesis of polyesters . It interacts with diols to form polyesters, which have been thoroughly characterized and compared with their synthesized analogues . The nature of these interactions involves the formation of ester bonds during polyester synthesis.
Molecular Mechanism
The molecular mechanism of 2,4-Furandicarboxylic acid primarily involves its role in the synthesis of polyesters . . This process involves the formation of ester bonds, which is a common reaction in polymer chemistry.
Subcellular Localization
Given its role in the synthesis of polyesters, it might be localized in areas of the cell where such synthesis processes occur .
Propiedades
IUPAC Name |
furan-2,4-dicarboxylic acid | |
---|---|---|
Source | PubChem | |
URL | https://pubchem.ncbi.nlm.nih.gov | |
Description | Data deposited in or computed by PubChem | |
InChI |
InChI=1S/C6H4O5/c7-5(8)3-1-4(6(9)10)11-2-3/h1-2H,(H,7,8)(H,9,10) | |
Source | PubChem | |
URL | https://pubchem.ncbi.nlm.nih.gov | |
Description | Data deposited in or computed by PubChem | |
InChI Key |
JOTDFEIYNHTJHZ-UHFFFAOYSA-N | |
Source | PubChem | |
URL | https://pubchem.ncbi.nlm.nih.gov | |
Description | Data deposited in or computed by PubChem | |
Canonical SMILES |
C1=C(OC=C1C(=O)O)C(=O)O | |
Source | PubChem | |
URL | https://pubchem.ncbi.nlm.nih.gov | |
Description | Data deposited in or computed by PubChem | |
Molecular Formula |
C6H4O5 | |
Source | PubChem | |
URL | https://pubchem.ncbi.nlm.nih.gov | |
Description | Data deposited in or computed by PubChem | |
DSSTOX Substance ID |
DTXSID30624273 | |
Record name | Furan-2,4-dicarboxylic acid | |
Source | EPA DSSTox | |
URL | https://comptox.epa.gov/dashboard/DTXSID30624273 | |
Description | DSSTox provides a high quality public chemistry resource for supporting improved predictive toxicology. | |
Molecular Weight |
156.09 g/mol | |
Source | PubChem | |
URL | https://pubchem.ncbi.nlm.nih.gov | |
Description | Data deposited in or computed by PubChem | |
CAS RN |
4282-28-4 | |
Record name | Furan-2,4-dicarboxylic acid | |
Source | EPA DSSTox | |
URL | https://comptox.epa.gov/dashboard/DTXSID30624273 | |
Description | DSSTox provides a high quality public chemistry resource for supporting improved predictive toxicology. | |
Record name | furan-2,4-dicarboxylic acid | |
Source | European Chemicals Agency (ECHA) | |
URL | https://echa.europa.eu/information-on-chemicals | |
Description | The European Chemicals Agency (ECHA) is an agency of the European Union which is the driving force among regulatory authorities in implementing the EU's groundbreaking chemicals legislation for the benefit of human health and the environment as well as for innovation and competitiveness. | |
Explanation | Use of the information, documents and data from the ECHA website is subject to the terms and conditions of this Legal Notice, and subject to other binding limitations provided for under applicable law, the information, documents and data made available on the ECHA website may be reproduced, distributed and/or used, totally or in part, for non-commercial purposes provided that ECHA is acknowledged as the source: "Source: European Chemicals Agency, http://echa.europa.eu/". Such acknowledgement must be included in each copy of the material. ECHA permits and encourages organisations and individuals to create links to the ECHA website under the following cumulative conditions: Links can only be made to webpages that provide a link to the Legal Notice page. | |
Retrosynthesis Analysis
AI-Powered Synthesis Planning: Our tool employs the Template_relevance Pistachio, Template_relevance Bkms_metabolic, Template_relevance Pistachio_ringbreaker, Template_relevance Reaxys, Template_relevance Reaxys_biocatalysis model, leveraging a vast database of chemical reactions to predict feasible synthetic routes.
One-Step Synthesis Focus: Specifically designed for one-step synthesis, it provides concise and direct routes for your target compounds, streamlining the synthesis process.
Accurate Predictions: Utilizing the extensive PISTACHIO, BKMS_METABOLIC, PISTACHIO_RINGBREAKER, REAXYS, REAXYS_BIOCATALYSIS database, our tool offers high-accuracy predictions, reflecting the latest in chemical research and data.
Strategy Settings
Precursor scoring | Relevance Heuristic |
---|---|
Min. plausibility | 0.01 |
Model | Template_relevance |
Template Set | Pistachio/Bkms_metabolic/Pistachio_ringbreaker/Reaxys/Reaxys_biocatalysis |
Top-N result to add to graph | 6 |
Feasible Synthetic Routes
Q & A
Q1: How does the structure of 2,4-FDCA influence the properties of 2,4-PEF compared to its more common isomer, poly(ethylene 2,5-furanoate) (2,5-PEF)?
A1: While both 2,5-FDCA and 2,4-FDCA can form polyesters with ethylene glycol, their structural differences significantly impact the resulting polymer properties. 2,5-FDCA leads to a more symmetrical polymer backbone in 2,5-PEF, allowing for efficient chain packing and promoting crystallinity. [] In contrast, the asymmetric nature of 2,4-FDCA disrupts this ordered arrangement in 2,4-PEF. [] This asymmetry hinders the formation of the periodic interchain C–H···O contacts typically observed in crystalline polymers. [] As a result, 2,4-PEF predominantly forms randomly coiled chains with gauche-ethylene glycol segments, favoring an amorphous structure. []
Q2: Can 2,4-FDCA be incorporated into other polyesters besides 2,4-PEF?
A2: Yes, research indicates that 2,4-FDCA can be copolymerized with other monomers to create copolyesters with tailored properties. For instance, 2,4-FDCA has been successfully copolymerized with terephthalic acid and ethylene glycol to produce transparent films of poly(ethylene terephthalate)-co-(ethylene 2,4-furandicarboxylate)s (PET-co-2,4-PEFs). [] This demonstrates the potential of using 2,4-FDCA as a building block in various copolyesters, potentially opening doors for new materials with specific functionalities.
Q3: What are the potential environmental benefits of utilizing 2,4-FDCA in polymer production?
A3: 2,4-FDCA holds promise as a bio-based alternative to petroleum-derived monomers commonly used in polyester synthesis. Its production from renewable resources like carbohydrates offers a more sustainable approach compared to conventional fossil fuel-based methods. [] Utilizing 2,4-FDCA in polymers aligns with the growing demand for eco-friendly materials and could contribute to a circular economy by reducing reliance on finite resources. Further research into efficient and scalable production methods for 2,4-FDCA is crucial to fully realize its environmental benefits.
Descargo de responsabilidad e información sobre productos de investigación in vitro
Tenga en cuenta que todos los artículos e información de productos presentados en BenchChem están destinados únicamente con fines informativos. Los productos disponibles para la compra en BenchChem están diseñados específicamente para estudios in vitro, que se realizan fuera de organismos vivos. Los estudios in vitro, derivados del término latino "in vidrio", involucran experimentos realizados en entornos de laboratorio controlados utilizando células o tejidos. Es importante tener en cuenta que estos productos no se clasifican como medicamentos y no han recibido la aprobación de la FDA para la prevención, tratamiento o cura de ninguna condición médica, dolencia o enfermedad. Debemos enfatizar que cualquier forma de introducción corporal de estos productos en humanos o animales está estrictamente prohibida por ley. Es esencial adherirse a estas pautas para garantizar el cumplimiento de los estándares legales y éticos en la investigación y experimentación.