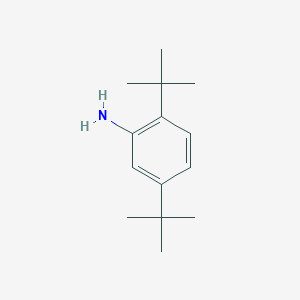
2,5-di-tert-Butylaniline
Descripción general
Descripción
2,5-di-tert-Butylaniline is an organic compound with the chemical formula C14H23N. It is a derivative of aniline, where two tert-butyl groups are substituted at the 2 and 5 positions of the benzene ring. This compound is known for its yellow to yellow-brown solid appearance and is soluble in organic solvents such as ether, benzene, and acetone .
Métodos De Preparación
Synthetic Routes and Reaction Conditions: 2,5-di-tert-Butylaniline is typically synthesized through the reaction of aniline with tert-butyl bromide in the presence of a base. The reaction is carried out under an inert atmosphere to prevent oxidation. The intermediate product formed is then treated with an acid to yield the final compound .
Industrial Production Methods: In industrial settings, the production of this compound follows a similar synthetic route but on a larger scale. The process involves the use of large reactors and precise control of reaction conditions to ensure high yield and purity. The final product is often purified through recrystallization from ethanol to obtain fine needles .
Análisis De Reacciones Químicas
2,5-di-tert-Butylaniline undergoes various chemical reactions, including:
Oxidation: This compound can be oxidized to form corresponding quinones or other oxidized derivatives. Common oxidizing agents include potassium permanganate and chromium trioxide.
Reduction: Reduction reactions can convert this compound to its corresponding amine derivatives. Typical reducing agents include lithium aluminum hydride and sodium borohydride.
Substitution: The compound can undergo electrophilic substitution reactions, where the tert-butyl groups provide steric hindrance, influencing the regioselectivity of the reaction. Common reagents include halogens and sulfonyl chlorides.
Major Products: The major products formed from these reactions depend on the specific conditions and reagents used. For example, oxidation may yield quinones, while substitution reactions can produce halogenated or sulfonated derivatives .
Aplicaciones Científicas De Investigación
2,5-di-tert-Butylaniline has several applications in scientific research:
Chemistry: It is used in the synthesis of rigid bidentate nitrogen ligands, such as 1,2-bis[(2,5-di-tert-butylphenyl)imino]acenaphthene (dtb-BIAN). These ligands are important in coordination chemistry and catalysis .
Biology and Medicine: While specific biological applications are less common, derivatives of this compound may be explored for their potential biological activity and as intermediates in the synthesis of pharmaceuticals.
Industry: The compound is used as a flame retardant additive in plastics and rubber materials to enhance their fire resistance .
Mecanismo De Acción
The mechanism of action of 2,5-di-tert-Butylaniline in its various applications depends on its chemical structure. In coordination chemistry, the tert-butyl groups provide steric hindrance, which can influence the binding properties of the ligands. The aniline moiety can participate in hydrogen bonding and other interactions, affecting the overall reactivity and stability of the compound .
Comparación Con Compuestos Similares
- 2-tert-Butylaniline
- 4-tert-Butylaniline
- 2,4,6-Tri-tert-butylaniline
Comparison: 2,5-di-tert-Butylaniline is unique due to the specific positioning of the tert-butyl groups, which provides distinct steric and electronic properties. Compared to 2-tert-Butylaniline and 4-tert-Butylaniline, the 2,5-substitution pattern offers different reactivity and selectivity in chemical reactions. The presence of two tert-butyl groups also enhances the compound’s solubility in organic solvents and its stability .
Actividad Biológica
2,5-Di-tert-butylaniline (DTBA) is a chemical compound with significant biological activity, primarily related to its interactions with biological macromolecules and its potential toxicity. This article delves into the various aspects of DTBA's biological activity, including its mechanisms of action, effects on cellular processes, and implications for health and safety.
Chemical Structure and Properties
This compound is characterized by the presence of two tert-butyl groups at the 2 and 5 positions of the aniline structure. Its molecular formula is , with a molecular weight of approximately 219.35 g/mol. The bulky tert-butyl groups contribute to steric hindrance, influencing its reactivity and interactions with other molecules.
Interaction with DNA
Research indicates that DTBA can form lesions on DNA, particularly targeting thymine bases. These lesions may lead to mutations if not adequately repaired, highlighting the compound's potential genotoxicity. Such interactions suggest that DTBA could pose risks in environments where it is prevalent, such as industrial settings or laboratories.
Enzyme Inhibition
DTBA has been shown to inhibit the activity of various enzymes, including acetylcholinesterase (AChE). This inhibition can disrupt neurotransmission and lead to neurotoxic effects. The compound's interaction with cytochrome P450 enzymes also suggests a role in the metabolism of xenobiotics, potentially leading to the formation of reactive intermediates that can cause oxidative stress.
Oxidative Stress
DTBA influences cellular signaling pathways related to oxidative stress responses. It has been observed to induce the expression of antioxidant genes, such as glutathione S-transferase, which are crucial for cellular defense against oxidative damage. Prolonged exposure to DTBA can result in persistent oxidative stress, contributing to cellular dysfunction and toxicity.
Toxicity Studies
In animal models, DTBA exhibits dose-dependent toxicity. At low doses, it may have minimal effects; however, at higher doses, it can induce significant hepatotoxicity and neurotoxicity. Studies have demonstrated that high concentrations lead to severe oxidative damage and disruption of normal cellular processes.
Case Study: Genotoxicity Assessment
A study assessing the genotoxic effects of DTBA involved exposing cultured mammalian cells to varying concentrations of the compound. The results indicated a dose-dependent increase in DNA damage as measured by comet assays, confirming its potential as a genotoxic agent.
Concentration (µM) | DNA Damage (Comet Score) |
---|---|
0 | 0 |
10 | 1 |
50 | 3 |
100 | 5 |
Pharmacokinetics
The pharmacokinetic profile of DTBA reveals that it is rapidly absorbed and distributed within tissues due to its lipophilicity. The compound's interaction with transport proteins facilitates its movement across cellular membranes, influencing its biological activity and potential toxicity.
Propiedades
IUPAC Name |
2,5-ditert-butylaniline | |
---|---|---|
Source | PubChem | |
URL | https://pubchem.ncbi.nlm.nih.gov | |
Description | Data deposited in or computed by PubChem | |
InChI |
InChI=1S/C14H23N/c1-13(2,3)10-7-8-11(12(15)9-10)14(4,5)6/h7-9H,15H2,1-6H3 | |
Source | PubChem | |
URL | https://pubchem.ncbi.nlm.nih.gov | |
Description | Data deposited in or computed by PubChem | |
InChI Key |
NUZVLYNISQOZOW-UHFFFAOYSA-N | |
Source | PubChem | |
URL | https://pubchem.ncbi.nlm.nih.gov | |
Description | Data deposited in or computed by PubChem | |
Canonical SMILES |
CC(C)(C)C1=CC(=C(C=C1)C(C)(C)C)N | |
Source | PubChem | |
URL | https://pubchem.ncbi.nlm.nih.gov | |
Description | Data deposited in or computed by PubChem | |
Molecular Formula |
C14H23N | |
Source | PubChem | |
URL | https://pubchem.ncbi.nlm.nih.gov | |
Description | Data deposited in or computed by PubChem | |
DSSTOX Substance ID |
DTXSID50345559 | |
Record name | 2,5-di-tert-Butylaniline | |
Source | EPA DSSTox | |
URL | https://comptox.epa.gov/dashboard/DTXSID50345559 | |
Description | DSSTox provides a high quality public chemistry resource for supporting improved predictive toxicology. | |
Molecular Weight |
205.34 g/mol | |
Source | PubChem | |
URL | https://pubchem.ncbi.nlm.nih.gov | |
Description | Data deposited in or computed by PubChem | |
CAS No. |
21860-03-7 | |
Record name | 2,5-di-tert-Butylaniline | |
Source | EPA DSSTox | |
URL | https://comptox.epa.gov/dashboard/DTXSID50345559 | |
Description | DSSTox provides a high quality public chemistry resource for supporting improved predictive toxicology. | |
Record name | Benzenamine, 2,5-bis(1,1-dimethylethyl)- | |
Source | European Chemicals Agency (ECHA) | |
URL | https://echa.europa.eu/information-on-chemicals | |
Description | The European Chemicals Agency (ECHA) is an agency of the European Union which is the driving force among regulatory authorities in implementing the EU's groundbreaking chemicals legislation for the benefit of human health and the environment as well as for innovation and competitiveness. | |
Explanation | Use of the information, documents and data from the ECHA website is subject to the terms and conditions of this Legal Notice, and subject to other binding limitations provided for under applicable law, the information, documents and data made available on the ECHA website may be reproduced, distributed and/or used, totally or in part, for non-commercial purposes provided that ECHA is acknowledged as the source: "Source: European Chemicals Agency, http://echa.europa.eu/". Such acknowledgement must be included in each copy of the material. ECHA permits and encourages organisations and individuals to create links to the ECHA website under the following cumulative conditions: Links can only be made to webpages that provide a link to the Legal Notice page. | |
Retrosynthesis Analysis
AI-Powered Synthesis Planning: Our tool employs the Template_relevance Pistachio, Template_relevance Bkms_metabolic, Template_relevance Pistachio_ringbreaker, Template_relevance Reaxys, Template_relevance Reaxys_biocatalysis model, leveraging a vast database of chemical reactions to predict feasible synthetic routes.
One-Step Synthesis Focus: Specifically designed for one-step synthesis, it provides concise and direct routes for your target compounds, streamlining the synthesis process.
Accurate Predictions: Utilizing the extensive PISTACHIO, BKMS_METABOLIC, PISTACHIO_RINGBREAKER, REAXYS, REAXYS_BIOCATALYSIS database, our tool offers high-accuracy predictions, reflecting the latest in chemical research and data.
Strategy Settings
Precursor scoring | Relevance Heuristic |
---|---|
Min. plausibility | 0.01 |
Model | Template_relevance |
Template Set | Pistachio/Bkms_metabolic/Pistachio_ringbreaker/Reaxys/Reaxys_biocatalysis |
Top-N result to add to graph | 6 |
Feasible Synthetic Routes
Q1: What is the role of 2,5-di-tert-butylaniline in photochemical reactions involving quinones?
A1: this compound acts as an electron donor in photoinduced electron transfer reactions with quinones, like 2-methyl-1,4-naphthoquinone. [] Upon excitation, the triplet state of the quinone accepts an electron from the amine, resulting in the formation of a radical ion pair. [] The presence of salts like lithium perchlorate can significantly influence the subsequent reactions of these radical ion pairs, promoting the formation of free radical ions and affecting their recombination kinetics. []
Q2: How does this compound react with alkylzinc complexes bearing a bulky amido-imino ligand?
A2: Instead of undergoing the expected alkane elimination, this compound acts as a proton source when reacting with the alkylzinc complex (L)Zn-nBu, where L represents the bulky amido-imino ligand [1-n-butyl-2-(2,6-diisopropylphenyl)iminoacenaphthen-1-yl]-2,6-diisopropylphenylamide. [] This protonation leads to the formation of the corresponding amino-imine LH and a zinc species. [] This unusual reactivity highlights the significant influence of steric hindrance imposed by the bulky ligand on the reactivity of the zinc center.
Descargo de responsabilidad e información sobre productos de investigación in vitro
Tenga en cuenta que todos los artículos e información de productos presentados en BenchChem están destinados únicamente con fines informativos. Los productos disponibles para la compra en BenchChem están diseñados específicamente para estudios in vitro, que se realizan fuera de organismos vivos. Los estudios in vitro, derivados del término latino "in vidrio", involucran experimentos realizados en entornos de laboratorio controlados utilizando células o tejidos. Es importante tener en cuenta que estos productos no se clasifican como medicamentos y no han recibido la aprobación de la FDA para la prevención, tratamiento o cura de ninguna condición médica, dolencia o enfermedad. Debemos enfatizar que cualquier forma de introducción corporal de estos productos en humanos o animales está estrictamente prohibida por ley. Es esencial adherirse a estas pautas para garantizar el cumplimiento de los estándares legales y éticos en la investigación y experimentación.