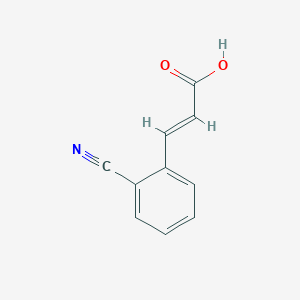
2-Cyanocinnamic acid
Descripción general
Descripción
2-Cyanocinnamic acid is a compound with the molecular formula C10H7NO2 . It is a derivative of cinnamic acid, which is a natural product found in high levels of plant-based foods .
Synthesis Analysis
Novel silyl cyanocinnamic acid derivatives have been synthesized and evaluated as potential anticancer agents . Structures of synthesized α-Cyanocinnamic acid derivatives have also been reported .
Molecular Structure Analysis
The molecular structure of 2-Cyanocinnamic acid consists of a cinnamoyl nucleus . The molecular formula is C10H7NO2, with an average mass of 173.168 Da and a monoisotopic mass of 173.047684 Da .
Physical And Chemical Properties Analysis
2-Cyanocinnamic acid has a density of 1.3±0.1 g/cm3, a boiling point of 383.9±25.0 °C at 760 mmHg, and a flash point of 186.0±23.2 °C . It has 3 H bond acceptors, 1 H bond donor, and 3 freely rotating bonds .
Aplicaciones Científicas De Investigación
Cancer Research
2-Cyanocinnamic acid has been used in cancer research, specifically in studies related to hyperthermic cytotoxicity . It has been found that when 2-Cyanocinnamic acid is administered simultaneously with oxidizing dyes like 2,3,5-triphenyltetrazolium chloride and methylene blue, it can greatly potentiate hyperthermic cytotoxicity . This means that it can enhance the ability of these compounds to kill cells at higher temperatures .
Anticancer Agents
Novel silyl cyanocinnamic acid derivatives have been synthesized and evaluated as potential anticancer agents . In vitro studies reveal that lead derivatives 2a and 2b have enhanced cancer cell proliferation inhibition properties when compared to the parent .
Chemical Role
According to ChEBI (Chemical Entities of Biological Interest), 2-Cyanocinnamic acid is classified as a Bronsted acid . A Bronsted acid is a molecular entity capable of donating a hydron to an acceptor (Bronsted base) .
Cinnamic Acid Derivative
2-Cyanocinnamic acid is a cinnamic acid derivative having a cyano substituent at the 2-position of the phenyl ring . This makes it a part of the larger family of cinnamic acids, which have a wide range of applications in the pharmaceutical and food industries .
Safety And Hazards
Propiedades
IUPAC Name |
(E)-3-(2-cyanophenyl)prop-2-enoic acid | |
---|---|---|
Source | PubChem | |
URL | https://pubchem.ncbi.nlm.nih.gov | |
Description | Data deposited in or computed by PubChem | |
InChI |
InChI=1S/C10H7NO2/c11-7-9-4-2-1-3-8(9)5-6-10(12)13/h1-6H,(H,12,13)/b6-5+ | |
Source | PubChem | |
URL | https://pubchem.ncbi.nlm.nih.gov | |
Description | Data deposited in or computed by PubChem | |
InChI Key |
HQVOPXGNHGTKOD-AATRIKPKSA-N | |
Source | PubChem | |
URL | https://pubchem.ncbi.nlm.nih.gov | |
Description | Data deposited in or computed by PubChem | |
Canonical SMILES |
C1=CC=C(C(=C1)C=CC(=O)O)C#N | |
Source | PubChem | |
URL | https://pubchem.ncbi.nlm.nih.gov | |
Description | Data deposited in or computed by PubChem | |
Isomeric SMILES |
C1=CC=C(C(=C1)/C=C/C(=O)O)C#N | |
Source | PubChem | |
URL | https://pubchem.ncbi.nlm.nih.gov | |
Description | Data deposited in or computed by PubChem | |
Molecular Formula |
C10H7NO2 | |
Source | PubChem | |
URL | https://pubchem.ncbi.nlm.nih.gov | |
Description | Data deposited in or computed by PubChem | |
Molecular Weight |
173.17 g/mol | |
Source | PubChem | |
URL | https://pubchem.ncbi.nlm.nih.gov | |
Description | Data deposited in or computed by PubChem | |
Product Name |
2-Cyanocinnamic acid | |
CAS RN |
61147-65-7 | |
Record name | NSC100172 | |
Source | DTP/NCI | |
URL | https://dtp.cancer.gov/dtpstandard/servlet/dwindex?searchtype=NSC&outputformat=html&searchlist=100172 | |
Description | The NCI Development Therapeutics Program (DTP) provides services and resources to the academic and private-sector research communities worldwide to facilitate the discovery and development of new cancer therapeutic agents. | |
Explanation | Unless otherwise indicated, all text within NCI products is free of copyright and may be reused without our permission. Credit the National Cancer Institute as the source. | |
Retrosynthesis Analysis
AI-Powered Synthesis Planning: Our tool employs the Template_relevance Pistachio, Template_relevance Bkms_metabolic, Template_relevance Pistachio_ringbreaker, Template_relevance Reaxys, Template_relevance Reaxys_biocatalysis model, leveraging a vast database of chemical reactions to predict feasible synthetic routes.
One-Step Synthesis Focus: Specifically designed for one-step synthesis, it provides concise and direct routes for your target compounds, streamlining the synthesis process.
Accurate Predictions: Utilizing the extensive PISTACHIO, BKMS_METABOLIC, PISTACHIO_RINGBREAKER, REAXYS, REAXYS_BIOCATALYSIS database, our tool offers high-accuracy predictions, reflecting the latest in chemical research and data.
Strategy Settings
Precursor scoring | Relevance Heuristic |
---|---|
Min. plausibility | 0.01 |
Model | Template_relevance |
Template Set | Pistachio/Bkms_metabolic/Pistachio_ringbreaker/Reaxys/Reaxys_biocatalysis |
Top-N result to add to graph | 6 |
Feasible Synthetic Routes
Q & A
Q1: How does 2-cyanocinnamic acid impact cell survival, particularly in the context of hyperthermia?
A1: 2-Cyanocinnamic acid acts as an inhibitor of mitochondrial pyruvate transport [, ]. This inhibition disrupts cellular energy production, particularly under hyperthermic conditions (elevated temperatures). Research suggests that pyruvate plays a protective role against hyperthermic damage []. By inhibiting pyruvate transport, 2-cyanocinnamic acid sensitizes cells to hyperthermia, leading to increased cell death at even slightly elevated temperatures, such as 41°C [].
Q2: Can the cytotoxic effects of 2-cyanocinnamic acid be enhanced?
A2: Yes, studies have shown that certain oxidizing dyes, like 2,3,5-triphenyltetrazolium chloride and methylene blue, significantly enhance the hyperthermic cytotoxicity of 2-cyanocinnamic acid when administered concurrently []. This synergistic effect leads to greater cell death compared to either compound alone at elevated temperatures. Interestingly, these dyes alone or in combination with 2-cyanocinnamic acid at normal physiological temperatures do not exhibit significant toxicity []. This suggests a mechanism potentially linked to the depletion of NAD(P)H, a crucial coenzyme in cellular redox reactions, under hyperthermic conditions [].
Q3: Besides its role in cytotoxicity, are there other cellular processes that 2-cyanocinnamic acid influences?
A3: Research indicates that 2-cyanocinnamic acid can inhibit heat shock protein (HSP) synthesis under certain conditions []. HSPs are crucial for cellular protection and repair mechanisms, particularly in response to stress like heat shock. This inhibition further points to the potential role of pyruvate in regulating cellular stress responses and highlights the multifaceted effects of 2-cyanocinnamic acid on cellular processes [].
Q4: Beyond cytotoxicity, are there other applications of 2-cyanocinnamic acid derivatives?
A4: Yes, chiral derivatives of 2-cyanocinnamic acid have shown promise in asymmetric Diels-Alder reactions []. These reactions are powerful tools in organic synthesis, allowing for the creation of complex molecules with high enantioselectivity. Specifically, (E)-2-cyanocinnamates derived from (S)-ethyl lactate and (R)-pantolactone, when reacted with cyclopentadiene in the presence of a titanium tetrachloride catalyst, yield enantiomerically pure cycloadducts []. This highlights the potential of 2-cyanocinnamic acid derivatives as versatile building blocks in asymmetric synthesis.
Descargo de responsabilidad e información sobre productos de investigación in vitro
Tenga en cuenta que todos los artículos e información de productos presentados en BenchChem están destinados únicamente con fines informativos. Los productos disponibles para la compra en BenchChem están diseñados específicamente para estudios in vitro, que se realizan fuera de organismos vivos. Los estudios in vitro, derivados del término latino "in vidrio", involucran experimentos realizados en entornos de laboratorio controlados utilizando células o tejidos. Es importante tener en cuenta que estos productos no se clasifican como medicamentos y no han recibido la aprobación de la FDA para la prevención, tratamiento o cura de ninguna condición médica, dolencia o enfermedad. Debemos enfatizar que cualquier forma de introducción corporal de estos productos en humanos o animales está estrictamente prohibida por ley. Es esencial adherirse a estas pautas para garantizar el cumplimiento de los estándares legales y éticos en la investigación y experimentación.