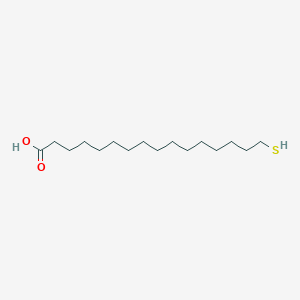
16-Mercaptohexadecanoic acid
Descripción general
Descripción
16-Mercaptohexadecanoic acid (MHA) is an alkanethiol with long-chained −CH2 groups . It forms a self-assembled monolayer (SAM) on a wide range of substrates .
Synthesis Analysis
16-Mercaptohexadecanoic acid capped gold nanoparticles have been synthesized through a single-phase synthesis method . It has also been used as reductants and ligands to synthesize fluorescent Au NCs .Molecular Structure Analysis
The molecular structure of 16-Mercaptohexadecanoic acid can be represented by the formula C16H32O2S .Chemical Reactions Analysis
The inner surfaces of a nanoporous carbon are modified by 16-Mercaptohexadecanoic acid . The degree of wettability is electrically controllable: by applying a negative potential difference across the solid-liquid interface, the ion transport pressure in the nanopores can be considerably lowered .Physical And Chemical Properties Analysis
16-Mercaptohexadecanoic acid has a melting point of 65-69 °C and a density of 0.787 g/mL at 25 °C .Aplicaciones Científicas De Investigación
Surface Modification for Enhanced Raman Spectroscopy
MHA can form self-assembled monolayers (SAMs) on gold surfaces, which are utilized in the fabrication of nanoresonators for shell-isolated nanoparticle enhanced Raman spectroscopy (SHINERS) . This application is significant in bio-engineering and chemical sensing .
Electrochemical Sensing
Gold electrodes modified with MHA can be used for the sensitive detection of clozapine , an antipsychotic medication. This modification enhances the electrode’s sensitivity and specificity .
Wettability Control in Nanoporous Carbon
The inner surfaces of nanoporous carbon modified with MHA exhibit electrically controllable wettability. By applying a negative potential difference, the ion transport pressure in the nanopores can be significantly reduced, which is valuable for controlled liquid transport and filtration processes .
Bioimaging Probes
MHA is used as a templating agent to produce photoluminescent nanoclusters, which emit light in various colors. These nanoclusters serve as probes for bioimaging applications , aiding in the visualization of biological processes .
Mecanismo De Acción
Target of Action
16-Mercaptohexadecanoic acid (MHA) is a long-chained alkanethiol . It primarily targets a variety of surfaces to form self-assembled monolayers (SAMs) . These surfaces include gold dipyramids and gold electrodes .
Mode of Action
MHA interacts with its targets by forming SAMs . This interaction aids in the creation of biosensors and nanoelectronic devices . It can also be used to surface modify gold electrodes for sensitive detection of certain compounds .
Biochemical Pathways
MHA is integral to the study of enzyme kinetics and cellular signaling due to its interaction with proteins and enzymes . It interacts with cytochrome P450 enzymes involved in metabolic pathways . Additionally, it binds to fatty acid-binding proteins, which are key in fatty acid transport and metabolism .
Pharmacokinetics
Its ability to form sams on various surfaces suggests that it may have unique distribution properties
Result of Action
The molecular and cellular effects of MHA’s action are primarily seen in its capacity to form SAMs on gold, aiding in the creation of biosensors and nanoelectronic devices . It also plays a role in enzyme kinetics and cellular signaling due to its interaction with proteins and enzymes .
Action Environment
The action of MHA can be influenced by environmental factors. For instance, the degree of wettability of MHA-modified nanoporous carbon surfaces is electrically controllable . By applying a negative potential difference across the solid-liquid interface, the ion transport pressure in the nanopores can be considerably lowered .
Safety and Hazards
16-Mercaptohexadecanoic acid should be handled with care to avoid contact with skin and eyes, and formation of dust and aerosols . It is recommended to use personal protective equipment and wear chemical impermeable gloves . In case of accidental ingestion or contact, immediate medical attention is required .
Direcciones Futuras
The modification of 16-Mercaptohexadecanoic acid on liquid transport in a nanoporous carbon has been studied . This could open up new possibilities for the use of 16-Mercaptohexadecanoic acid in the fabrication of nanoresonators for shell-isolated nanoparticle enhanced Raman spectroscopy (SHINERS) .
Relevant Papers Several papers have been published on 16-Mercaptohexadecanoic acid. For instance, a study on the effect of 16-Mercaptohexadecanoic acid modification on liquid transport in a nanoporous carbon , and another on the formation of an α-cyclodextrin/16-Mercaptohexadecanoic acid complex and its deposition on gold surfaces .
Propiedades
IUPAC Name |
16-sulfanylhexadecanoic acid | |
---|---|---|
Source | PubChem | |
URL | https://pubchem.ncbi.nlm.nih.gov | |
Description | Data deposited in or computed by PubChem | |
InChI |
InChI=1S/C16H32O2S/c17-16(18)14-12-10-8-6-4-2-1-3-5-7-9-11-13-15-19/h19H,1-15H2,(H,17,18) | |
Source | PubChem | |
URL | https://pubchem.ncbi.nlm.nih.gov | |
Description | Data deposited in or computed by PubChem | |
InChI Key |
INOAASCWQMFJQA-UHFFFAOYSA-N | |
Source | PubChem | |
URL | https://pubchem.ncbi.nlm.nih.gov | |
Description | Data deposited in or computed by PubChem | |
Canonical SMILES |
C(CCCCCCCC(=O)O)CCCCCCCS | |
Source | PubChem | |
URL | https://pubchem.ncbi.nlm.nih.gov | |
Description | Data deposited in or computed by PubChem | |
Molecular Formula |
C16H32O2S | |
Source | PubChem | |
URL | https://pubchem.ncbi.nlm.nih.gov | |
Description | Data deposited in or computed by PubChem | |
DSSTOX Substance ID |
DTXSID00393287 | |
Record name | 16-Mercaptohexadecanoic acid | |
Source | EPA DSSTox | |
URL | https://comptox.epa.gov/dashboard/DTXSID00393287 | |
Description | DSSTox provides a high quality public chemistry resource for supporting improved predictive toxicology. | |
Molecular Weight |
288.5 g/mol | |
Source | PubChem | |
URL | https://pubchem.ncbi.nlm.nih.gov | |
Description | Data deposited in or computed by PubChem | |
CAS RN |
69839-68-5 | |
Record name | 16-Mercaptohexadecanoic acid | |
Source | EPA DSSTox | |
URL | https://comptox.epa.gov/dashboard/DTXSID00393287 | |
Description | DSSTox provides a high quality public chemistry resource for supporting improved predictive toxicology. | |
Record name | 16-Sulfanylhexadecanoic acid | |
Source | European Chemicals Agency (ECHA) | |
URL | https://echa.europa.eu/information-on-chemicals | |
Description | The European Chemicals Agency (ECHA) is an agency of the European Union which is the driving force among regulatory authorities in implementing the EU's groundbreaking chemicals legislation for the benefit of human health and the environment as well as for innovation and competitiveness. | |
Explanation | Use of the information, documents and data from the ECHA website is subject to the terms and conditions of this Legal Notice, and subject to other binding limitations provided for under applicable law, the information, documents and data made available on the ECHA website may be reproduced, distributed and/or used, totally or in part, for non-commercial purposes provided that ECHA is acknowledged as the source: "Source: European Chemicals Agency, http://echa.europa.eu/". Such acknowledgement must be included in each copy of the material. ECHA permits and encourages organisations and individuals to create links to the ECHA website under the following cumulative conditions: Links can only be made to webpages that provide a link to the Legal Notice page. | |
Synthesis routes and methods I
Procedure details
Synthesis routes and methods II
Procedure details
Retrosynthesis Analysis
AI-Powered Synthesis Planning: Our tool employs the Template_relevance Pistachio, Template_relevance Bkms_metabolic, Template_relevance Pistachio_ringbreaker, Template_relevance Reaxys, Template_relevance Reaxys_biocatalysis model, leveraging a vast database of chemical reactions to predict feasible synthetic routes.
One-Step Synthesis Focus: Specifically designed for one-step synthesis, it provides concise and direct routes for your target compounds, streamlining the synthesis process.
Accurate Predictions: Utilizing the extensive PISTACHIO, BKMS_METABOLIC, PISTACHIO_RINGBREAKER, REAXYS, REAXYS_BIOCATALYSIS database, our tool offers high-accuracy predictions, reflecting the latest in chemical research and data.
Strategy Settings
Precursor scoring | Relevance Heuristic |
---|---|
Min. plausibility | 0.01 |
Model | Template_relevance |
Template Set | Pistachio/Bkms_metabolic/Pistachio_ringbreaker/Reaxys/Reaxys_biocatalysis |
Top-N result to add to graph | 6 |
Feasible Synthetic Routes
Descargo de responsabilidad e información sobre productos de investigación in vitro
Tenga en cuenta que todos los artículos e información de productos presentados en BenchChem están destinados únicamente con fines informativos. Los productos disponibles para la compra en BenchChem están diseñados específicamente para estudios in vitro, que se realizan fuera de organismos vivos. Los estudios in vitro, derivados del término latino "in vidrio", involucran experimentos realizados en entornos de laboratorio controlados utilizando células o tejidos. Es importante tener en cuenta que estos productos no se clasifican como medicamentos y no han recibido la aprobación de la FDA para la prevención, tratamiento o cura de ninguna condición médica, dolencia o enfermedad. Debemos enfatizar que cualquier forma de introducción corporal de estos productos en humanos o animales está estrictamente prohibida por ley. Es esencial adherirse a estas pautas para garantizar el cumplimiento de los estándares legales y éticos en la investigación y experimentación.