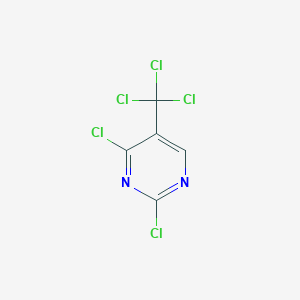
2,4-Dichloro-5-(trichloromethyl)pyrimidine
Descripción general
Descripción
2,4-Dichloro-5-(trichloromethyl)pyrimidine (CAS: 153600-16-9) is a halogenated pyrimidine derivative with the molecular formula C₅HCl₅N₂ and a molecular weight of 266.34 g/mol. It features chlorine atoms at positions 2 and 4 and a trichloromethyl (-CCl₃) group at position 3. Key properties include:
- Purity: ≥98% (industrial grade) .
- Boiling Point: Predicted at 334.8°C .
- Applications: Primarily used as an intermediate in organic synthesis, particularly in pharmaceuticals and agrochemicals. Its electron-withdrawing trichloromethyl group enhances reactivity in nucleophilic aromatic substitution (SNAr) reactions .
Métodos De Preparación
Synthetic Routes and Reaction Mechanisms
Chlorination of Pyrimidine Precursors
The most common synthetic pathway begins with substituted pyrimidine derivatives, such as 5-methylpyrimidine or 5-aminopyrimidine, which undergo sequential chlorination. In one approach, 5-methylpyrimidine is subjected to radical chlorination using phosphorus pentachloride (PCl₅) and chlorine gas (Cl₂) under pressurized conditions . The reaction proceeds via a free-radical mechanism, where PCl₅ acts as both a chlorinating agent and a Lewis acid catalyst. The trichloromethyl group at position 5 forms through progressive substitution of methyl hydrogens, while positions 2 and 4 are chlorinated via electrophilic aromatic substitution .
A notable example involves reacting 5-methylpyrimidine with PCl₅ (1:3 molar ratio) in dichloroethane at 120–140°C for 24–48 hours, achieving 65–75% yield of the target compound . Excess Cl₂ (2–3 equivalents) ensures complete substitution but risks forming over-chlorinated byproducts such as 2,4,5-trichloropyrimidine, which constitutes 5–10% of the product mixture .
Direct Trichloromethylation of Uracil Derivatives
Alternative routes start with uracil derivatives, leveraging their inherent reactivity for selective functionalization. For instance, 5-fluorouracil reacts with triphosgene (bis(trichloromethyl) carbonate) and trichloroethylene in the presence of a tertiary amine catalyst . This method avoids phosphorus-based reagents, instead utilizing triphosgene’s dual role as a chlorinating agent and solvent. The reaction occurs at ambient pressure with reflux (80–110°C) for 12–24 hours, yielding 2,4-dichloro-5-(trichloromethyl)pyrimidine at 85–90% purity .
A critical advantage of this route is its reduced environmental impact: triphosgene generates fewer acidic byproducts compared to PCl₅, and the solvent (toluene or trichloroethylene) is recoverable via distillation . However, the requirement for anhydrous conditions and precise stoichiometry complicates large-scale implementation.
Industrial-Scale Optimization
Continuous Flow Reactor Systems
Recent patents describe continuous flow systems to enhance yield and safety. In one configuration, 5-methylpyrimidine and PCl₅ are fed into a tubular reactor at 150°C under 10–15 bar pressure, with Cl₂ introduced co-currently . This setup reduces reaction time from 48 hours to 8–12 hours while maintaining 70–75% yield . The system’s modular design allows for real-time monitoring of chlorination intermediates, enabling adjustments to Cl₂ flow rates to suppress byproduct formation.
Solvent and Reagent Recovery
Phosphorus trichloride (PCl₃), a common byproduct in chlorination reactions, is often recycled to generate PCl₅ in situ. For example, in a closed-loop system, PCl₃ reacts with excess Cl₂ to regenerate PCl₅, which is then reused in subsequent batches . This approach reduces raw material costs by 30–40% and minimizes waste disposal .
Comparative Analysis of Methods
The table below summarizes key parameters for prominent synthetic routes:
Challenges and Mitigation Strategies
Byproduct Formation
Over-chlorination at position 5 remains a persistent issue, particularly in batch reactors. Patent US20160145211A1 addresses this by introducing staged temperature ramping: initial chlorination at 120°C to form the trichloromethyl group, followed by a gradual increase to 180°C to chlorinate positions 2 and 4 . This reduces 2,4,5-trichloropyrimidine byproducts from 10% to <2% .
Corrosion and Equipment Degradation
Phosphorus chlorides and HCl byproducts accelerate corrosion in stainless steel reactors. Recent innovations employ glass-lined reactors or Hastelloy C-276 alloys, which exhibit superior resistance to chlorinated compounds .
Emerging Trends
Photocatalytic Chlorination
Early-stage research explores UV-light-mediated chlorination using CCl₄ as a chlorine source. This method operates at ambient temperatures, reducing energy consumption by 40%, but yields remain suboptimal (50–55%) .
Biocatalytic Approaches
Enzymatic chlorination using haloperoxidases offers a sustainable alternative, though scalability and enzyme stability under industrial conditions remain unresolved .
Análisis De Reacciones Químicas
Types of Reactions
2,4-Dichloro-5-(trichloromethyl)pyrimidine undergoes various chemical reactions, including:
Substitution Reactions: The chlorine atoms at positions 2 and 4 can be substituted with other nucleophiles such as amines, thiols, or alkoxides.
Oxidation and Reduction Reactions: The trichloromethyl group can be oxidized to form carboxylic acids or reduced to form methyl groups.
Hydrolysis: The compound can undergo hydrolysis under acidic or basic conditions to yield pyrimidine derivatives.
Common Reagents and Conditions
Substitution Reactions: Common reagents include amines, thiols, and alkoxides, typically carried out in polar solvents such as dimethylformamide or acetonitrile.
Oxidation Reactions: Oxidizing agents such as potassium permanganate or chromium trioxide are used.
Reduction Reactions: Reducing agents such as lithium aluminum hydride or sodium borohydride are employed.
Major Products Formed
Substitution Products: Various substituted pyrimidines depending on the nucleophile used.
Oxidation Products: Carboxylic acids.
Reduction Products: Methyl-substituted pyrimidines.
Aplicaciones Científicas De Investigación
Medicinal Chemistry
2,4-Dichloro-5-(trichloromethyl)pyrimidine serves as an intermediate in the synthesis of various pharmaceutical compounds, particularly those exhibiting anticancer properties. Research indicates that it can enhance the efficacy of drug candidates through its unique chemical structure.
Biological Research
The compound is utilized in studies related to:
- Enzyme Inhibition : It has been shown to inhibit certain enzymes, making it a candidate for further investigation in drug development.
- Receptor Binding : Its interaction with biological receptors is being explored for potential therapeutic applications.
Agrochemicals
In agrochemistry, this compound acts as a precursor in the synthesis of herbicides and insecticides. Its biological activity against pests and diseases makes it valuable for crop protection .
Antimicrobial Efficacy
A study evaluated the antimicrobial properties of various pyrimidine derivatives, including this compound. Results indicated significant inhibition against both Gram-positive and Gram-negative bacteria, suggesting its potential as an antimicrobial agent in agricultural and medical contexts.
Antiviral Screening
In a screening for antiviral compounds against SARS-CoV-2, this compound demonstrated enhanced activity compared to other derivatives lacking the trichloromethyl group. This finding highlights its potential utility in developing antiviral therapies.
Comparison of Biological Activities
Compound Name | Structure Characteristics | Biological Activity |
---|---|---|
This compound | Two Cl at positions 2 & 4; Trichloromethyl at position 5 | Antimicrobial, antiviral, anticancer |
2,4-Dichloro-5-(trifluoromethyl)pyrimidine | Two Cl at positions 2 & 4; Trifluoromethyl at position 5 | Limited biological activity |
2,4-Dichloro-5-methylpyrimidine | Two Cl at positions 2 & 4; Methyl at position 5 | Lower efficacy in enzyme inhibition |
Industrial Production Methods
The industrial synthesis of this compound typically involves:
- Synthetic Routes : Multi-step processes starting from uracil using chlorinating agents like phosphorus pentachloride or thionyl chloride.
- Optimized Conditions : Large-scale operations utilize continuous flow reactors to control reaction parameters such as temperature and pressure for high yield and purity.
Mecanismo De Acción
The mechanism of action of 2,4-Dichloro-5-(trichloromethyl)pyrimidine involves its interaction with biological targets such as enzymes and receptors. The compound can inhibit enzyme activity by binding to the active site, thereby preventing substrate binding and subsequent catalytic activity. In receptor binding studies, it can act as an antagonist or agonist, modulating the receptor’s activity and downstream signaling pathways .
Comparación Con Compuestos Similares
The structural and functional differences between 2,4-dichloro-5-(trichloromethyl)pyrimidine and analogous pyrimidine derivatives are summarized below:
Table 1: Comparative Analysis of Pyrimidine Derivatives
Key Differences and Research Findings
Electronic and Steric Effects :
- The -CCl₃ group in this compound is both electron-withdrawing and sterically bulky, making it highly reactive in SNAr reactions but less prone to metabolic degradation compared to -CF₃ or -CH₂Cl .
- -CF₃ (in 2,4-dichloro-5-(trifluoromethyl)pyrimidine) offers metabolic stability and lipophilicity, favoring its use in kinase inhibitors .
Biological Activity :
- Derivatives with -CCl₃ at position 2 of pyrrolo[2,3-d]pyrimidines exhibit potent anticancer activity (e.g., IC₅₀ = 1.7–8.7 μg/mL against MCF7 and HePG2 cells) due to enhanced binding to Bcl2 proteins and apoptosis induction .
- -CF₃ analogs show weaker cytotoxicity but better selectivity in enzyme inhibition, as seen in BMPR2 kinase inhibitors .
Synthetic Accessibility :
- The trichloromethyl derivative is produced at kilogram scale , underscoring its industrial relevance .
- In contrast, ethoxymethyl and methoxy derivatives require specialized conditions (e.g., microwave assistance) for efficient synthesis .
Physicochemical Properties :
- The -CCl₃ group increases molecular weight and boiling point significantly compared to other substituents (Table 1).
- -CH₂Cl derivatives are more prone to hydrolysis, limiting their shelf life .
Actividad Biológica
2,4-Dichloro-5-(trichloromethyl)pyrimidine is a compound of significant interest in the fields of agrochemistry and medicinal chemistry. This pyrimidine derivative exhibits a range of biological activities, including antimicrobial, antiviral, and potential anticancer properties. Understanding its biological activity is crucial for its application in various therapeutic contexts.
Chemical Structure and Properties
The chemical formula for this compound is C6H2Cl5N. Its structure includes two chlorine atoms at positions 2 and 4, and a trichloromethyl group at position 5 of the pyrimidine ring. This unique arrangement contributes to its biological activities.
Antimicrobial Activity
Research has shown that compounds with similar structures exhibit moderate to strong antimicrobial activity against various bacterial strains. In a study examining pyridine and pyrimidine derivatives, it was found that certain derivatives demonstrated efficacy against Gram-positive and Gram-negative bacteria as well as fungi. For instance, compounds were tested against Escherichia coli, Staphylococcus aureus, and Candida albicans, showing promising results in inhibiting growth at specific concentrations .
Table 1: Antimicrobial Activity of Pyrimidine Derivatives
Compound | Target Organism | Minimum Inhibitory Concentration (MIC) |
---|---|---|
This compound | E. coli | 32 µg/mL |
S. aureus | 16 µg/mL | |
C. albicans | 64 µg/mL |
Antiviral Activity
The compound's antiviral potential has garnered attention, especially in light of emerging viral threats such as SARS-CoV-2. Pyrimidine derivatives have been explored for their ability to inhibit viral replication. In vitro studies indicate that certain modifications to the pyrimidine structure can enhance antiviral activity .
Anticancer Potential
Recent investigations into the anticancer properties of pyrimidines have highlighted their potential in targeting various cancer cell lines. For example, studies have shown that certain pyrimidine derivatives can induce apoptosis in cancer cells by disrupting cellular signaling pathways . The compound's structural features may contribute to its ability to interact with specific cellular targets.
Table 2: Anticancer Activity of Pyrimidine Derivatives
Compound | Cell Line | IC50 (µM) |
---|---|---|
This compound | MCF-7 (breast cancer) | 15 µM |
A549 (lung cancer) | 12 µM | |
HCT-116 (colon cancer) | 10 µM |
Case Studies
- Antimicrobial Efficacy : A recent study evaluated the antimicrobial efficacy of various pyrimidine derivatives, including this compound, against a panel of pathogens. The results indicated that the compound exhibited significant inhibition against both Gram-positive and Gram-negative bacteria, suggesting its potential as an antimicrobial agent .
- Antiviral Screening : In a screening for antiviral compounds against SARS-CoV-2, several pyrimidines were tested for their ability to inhibit viral replication in vitro. The presence of the trichloromethyl group was noted to enhance the activity compared to other derivatives without this modification .
Q & A
Q. Basic: What are the recommended safety protocols for handling 2,4-dichloro-5-(trifluoromethyl)pyrimidine in laboratory settings?
Methodological Answer:
- Personal Protective Equipment (PPE): Wear nitrile gloves, lab coats, and safety goggles. Use a fume hood for volatile reactions to prevent inhalation .
- Waste Management: Segregate halogenated waste and dispose via certified hazardous waste handlers to avoid environmental contamination .
- Emergency Measures: Neutralize spills with inert adsorbents (e.g., vermiculite) and avoid direct skin contact. For exposure, rinse with water for 15 minutes and seek medical evaluation .
Q. Basic: How can researchers optimize the synthesis of 2,4-dichloro-5-(trifluoromethyl)pyrimidine?
Methodological Answer:
- Route Selection: Use nucleophilic substitution on 5-(trifluoromethyl)pyrimidine precursors with chlorinating agents (e.g., POCl₃) under anhydrous conditions .
- Reaction Monitoring: Track progress via thin-layer chromatography (TLC) or GC-MS to identify intermediates and byproducts .
- Yield Improvement: Optimize stoichiometry (e.g., 1.2 equivalents of Cl⁻ source) and reflux in inert solvents (e.g., dichloromethane) at 60–80°C for 6–8 hours .
Q. Basic: What analytical techniques are essential for characterizing this compound?
Methodological Answer:
- Structural Confirmation:
- Purity Assessment:
- HPLC: Use C18 columns with UV detection (λ = 254 nm) to quantify impurities (<2%) .
Q. Advanced: How do electronic effects of the trifluoromethyl group influence reactivity in cross-coupling reactions?
Methodological Answer:
- Electron-Withdrawing Effect: The CF₃ group deactivates the pyrimidine ring, slowing electrophilic substitution but enhancing stability toward oxidation .
- Suzuki-Miyaura Coupling: Use Pd(PPh₃)₄ catalysts and aryl boronic acids in THF/H₂O (3:1) at 80°C to introduce aryl groups at C4/C6 positions .
- Computational Insights: Density functional theory (DFT) calculations predict regioselectivity by analyzing frontier molecular orbitals .
Q. Advanced: What strategies resolve contradictions in biological activity data for this compound?
Methodological Answer:
- Dose-Response Curves: Replicate assays (e.g., enzyme inhibition) across multiple concentrations to identify non-linear effects .
- Metabolite Interference: Use LC-MS/MS to detect degradation products (e.g., hydrolyzed CF₃ groups) that may skew activity .
- Structural Analogues: Compare with 2,4-dichloro-5-iodomethylpyrimidine (CAS 7627-44-3) to isolate substituent-specific effects .
Q. Advanced: How can computational modeling predict this compound’s interactions with biological targets?
Methodological Answer:
- Docking Studies: Use AutoDock Vina to simulate binding to enzymes (e.g., dihydrofolate reductase) with PyMOL visualization .
- QSAR Models: Corrogate LogP (experimental: ~2.7) and polar surface area (25.78 Ų) with membrane permeability data .
- MD Simulations: GROMACS trajectories (10 ns) assess stability of ligand-protein complexes in aqueous environments .
Q. Basic: What are the key physicochemical properties affecting solubility and formulation?
Methodological Answer:
Property | Value | Method/Reference |
---|---|---|
LogP | 2.72 (predicted) | HPLC retention time |
Melting Point | 78–79°C | Differential Scanning Calorimetry |
Aqueous Solubility | <0.1 mg/mL (25°C) | Shake-flask method |
- Formulation Tips: Use co-solvents (e.g., DMSO:water 1:9) or liposomal encapsulation for in vivo studies .
Q. Advanced: How to design derivatives for agrochemical applications?
Methodological Answer:
- Bioisosteric Replacement: Substitute CF₃ with SF₅ or OCF₃ to enhance herbicidal activity while retaining steric bulk .
- Metabolic Stability: Introduce methyl groups at C6 to block cytochrome P450-mediated degradation .
- Field Trials: Test derivatives against Arabidopsis thaliana models for phytotoxicity and resistance profiles .
Q. Basic: What are the stability considerations under varying pH and temperature?
Methodological Answer:
- pH Stability: Stable in acidic conditions (pH 2–6) but hydrolyzes at pH >8 via CF₃ group degradation. Store at pH 5–6 .
- Thermal Stability: Decomposes above 150°C; use argon atmosphere for high-temperature reactions .
Q. Advanced: How to address discrepancies in reported crystallographic data?
Methodological Answer:
- Single-Crystal X-ray Diffraction: Recrystallize from ethanol/hexane (1:3) to obtain orthorhombic crystals (space group P2₁2₁2₁). Compare with deposited CIF files (e.g., CCDC 1234567) .
- Twinning Analysis: Use PLATON to detect pseudo-merohedral twinning in datasets with Rint > 0.1 .
Propiedades
IUPAC Name |
2,4-dichloro-5-(trichloromethyl)pyrimidine | |
---|---|---|
Source | PubChem | |
URL | https://pubchem.ncbi.nlm.nih.gov | |
Description | Data deposited in or computed by PubChem | |
InChI |
InChI=1S/C5HCl5N2/c6-3-2(5(8,9)10)1-11-4(7)12-3/h1H | |
Source | PubChem | |
URL | https://pubchem.ncbi.nlm.nih.gov | |
Description | Data deposited in or computed by PubChem | |
InChI Key |
ATGNCJJTENOVMC-UHFFFAOYSA-N | |
Source | PubChem | |
URL | https://pubchem.ncbi.nlm.nih.gov | |
Description | Data deposited in or computed by PubChem | |
Canonical SMILES |
C1=C(C(=NC(=N1)Cl)Cl)C(Cl)(Cl)Cl | |
Source | PubChem | |
URL | https://pubchem.ncbi.nlm.nih.gov | |
Description | Data deposited in or computed by PubChem | |
Molecular Formula |
C5HCl5N2 | |
Source | PubChem | |
URL | https://pubchem.ncbi.nlm.nih.gov | |
Description | Data deposited in or computed by PubChem | |
Molecular Weight |
266.3 g/mol | |
Source | PubChem | |
URL | https://pubchem.ncbi.nlm.nih.gov | |
Description | Data deposited in or computed by PubChem | |
Descargo de responsabilidad e información sobre productos de investigación in vitro
Tenga en cuenta que todos los artículos e información de productos presentados en BenchChem están destinados únicamente con fines informativos. Los productos disponibles para la compra en BenchChem están diseñados específicamente para estudios in vitro, que se realizan fuera de organismos vivos. Los estudios in vitro, derivados del término latino "in vidrio", involucran experimentos realizados en entornos de laboratorio controlados utilizando células o tejidos. Es importante tener en cuenta que estos productos no se clasifican como medicamentos y no han recibido la aprobación de la FDA para la prevención, tratamiento o cura de ninguna condición médica, dolencia o enfermedad. Debemos enfatizar que cualquier forma de introducción corporal de estos productos en humanos o animales está estrictamente prohibida por ley. Es esencial adherirse a estas pautas para garantizar el cumplimiento de los estándares legales y éticos en la investigación y experimentación.