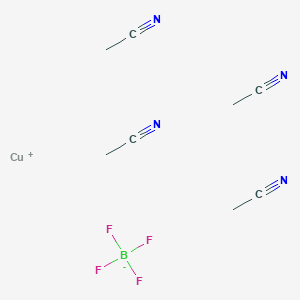
Tetrakis(acetonitrile)copper(I) tetrafluoroborate
Descripción general
Descripción
Tetrakis(acetonitrile)copper(I) tetrafluoroborate ([Cu(CH₃CN)₄]BF₄) is a cationic copper(I) complex with four acetonitrile ligands and a tetrafluoroborate (BF₄⁻) counterion. This compound is highly soluble in polar solvents such as acetonitrile, dimethyl sulfoxide (DMSO), and water, making it versatile in synthetic chemistry . Its weakly coordinating BF₄⁻ anion ensures labile acetonitrile ligands, facilitating ligand-exchange reactions critical for catalysis and material synthesis .
Key applications include:
- Catalysis: Used in enantioselective oxyarylation, [3+3]-cycloadditions, and methane-to-methanol conversion .
- Material Science: Precursor for metal-organic frameworks (MOFs) and coordination polymers .
- Biomedical Research: Drug delivery systems and antibacterial agents .
- Electrochemistry: CO₂ reduction to acetate and other C₂ products .
Métodos De Preparación
Traditional Synthetic Approaches
Modification of the Hexafluorophosphate Procedure
The earliest method for synthesizing [Cu(CH₃CN)₄]BF₄ adapts protocols developed for analogous hexafluorophosphate complexes. Copper(I) oxide (Cu₂O) reacts with tetrafluoroboric acid (HBF₄) in acetonitrile under inert conditions, yielding the target compound after recrystallization . Key steps include:
-
Reagents : Cu₂O, HBF₄ (48–50% in diethyl ether), anhydrous acetonitrile.
-
Conditions : Reaction conducted under nitrogen at 60°C for 4 hours.
-
Purification : Recrystallization from hot acetonitrile followed by drying under vacuum at 80°C for 12 hours .
This method achieves moderate yields (70–75%) but requires careful handling of moisture-sensitive reagents.
Copper(I) Tetrafluoroborate–Toluene Complex
An alternative route involves the reaction of preformed copper(I) tetrafluoroborate (CuBF₄) with acetonitrile in toluene . The CuBF₄–toluene adduct is prepared by treating copper powder with HBF₄ in toluene, followed by ligand exchange with acetonitrile:
-
Reaction : CuBF₄(toluene)₂ + 4 CH₃CN → [Cu(CH₃CN)₄]BF₄ + 2 toluene.
-
Yield : 80–85% after vacuum drying .
This method avoids Cu₂O but still relies on anhydrous solvents and inert atmospheres.
Boron Trifluoride Etherate Method
Copper(I) oxide reacts with boron trifluoride diethyl etherate (BF₃·OEt₂) in acetonitrile to generate [Cu(CH₃CN)₄]BF₄ :
-
Stoichiometry : Cu₂O + 2 BF₃·OEt₂ + 8 CH₃CN → 2 [Cu(CH₃CN)₄]BF₄ + H₂O + 2 Et₂O.
-
Conditions : Reflux for 2 hours under nitrogen.
-
Yield : 68–72% .
While effective, this method produces hazardous ether byproducts and requires rigorous exclusion of moisture.
Comproportionation Method
A comproportionation strategy employs copper(II) salts and metallic copper in refluxing acetonitrile :
-
Reaction : Cu(BF₄)₂ + Cu → 2 [Cu(CH₃CN)₄]BF₄.
-
Conditions : Reflux at 82°C for 6 hours under nitrogen.
-
Yield : 75–80% .
This method avoids Cu₂O but necessitates Cu(BF₄)₂, which is less commonly available than HBF₄.
Green One-Pot Synthesis in Aqueous Media
Recent advances prioritize sustainability by minimizing toxic solvents. A one-pot method uses copper(II) sulfate, ascorbic acid (vitamin C), and HBF₄ in water :
-
Reagents : CuSO₄·5H₂O, HBF₄, ascorbic acid, acetonitrile.
-
Mechanism : Ascorbic acid reduces Cu²⁺ to Cu⁺, which coordinates with acetonitrile.
-
Conditions : Room temperature, 1-hour reaction time.
Advantages Over Traditional Methods:
-
Solvent : Water replaces acetonitrile, reducing toxicity.
-
Safety : Eliminates BF₃·OEt₂ and anhydrous conditions.
-
Scalability : Suitable for gram-scale synthesis without specialized equipment .
Comparative Analysis of Synthetic Methods
Method | Reagents | Solvent | Yield | Environmental Impact |
---|---|---|---|---|
Hexafluorophosphate mod. | Cu₂O, HBF₄ | MeCN | 70–75% | High (toxic solvents) |
CuBF₄–toluene complex | Cu, HBF₄, toluene | Toluene/MeCN | 80–85% | Moderate |
BF₃·OEt₂ method | Cu₂O, BF₃·OEt₂ | MeCN | 68–72% | High (ether byproducts) |
Comproportionation | Cu(BF₄)₂, Cu | MeCN | 75–80% | Moderate |
Green one-pot | CuSO₄, ascorbic acid, HBF₄ | Water/MeCN | >80% | Low |
Análisis De Reacciones Químicas
Ligand Substitution Reactions
The acetonitrile ligands in [Cu(CH₃CN)₄]BF₄ are readily displaced by stronger-field ligands, enabling the synthesis of diverse copper complexes:
- Methoxyisobutyl isonitrile substitution :
Reaction with methoxyisobutyl isonitrile yields [Cu(CNC(CH₃)₂OCH₃)₄]BF₄, a precursor for technetium-99m radiopharmaceuticals .
Conditions : Carried out in acetonitrile at ambient temperature.
Diels-Alder Reactions
[Cu(CH₃CN)₄]BF₄ catalyzes [3+2]-cycloadditions between enoldiazo compounds and α-diazocarboximides, producing cyclopenta pyrrolo[2,1-b]oxazoles with >90% diastereoselectivity .
Ullmann Amination
The complex facilitates coupling of 1-halogenoanthraquinones (X = I, Br, Cl) with primary amines:
Halogen (X) | Reaction Rate | Major Product |
---|---|---|
Iodine | Fastest | Aminated anthraquinone |
Bromine | Moderate | Aminated anthraquinone |
Chlorine | Slowest | Dehalogenated byproduct |
Mechanism :
- Formation of Cu(I)-amine complex.
- Activation via proton abstraction.
- Generation of arylcopper(III) intermediate .
Aerobic Oxidation of Alcohols
Primary alcohols are oxidized to aldehydes under ambient air, with the catalyst enabling a "greener" pathway :
Example : Benzyl alcohol → Benzaldehyde (yield: 85–92%).
Mannich Reactions
With chiral phosphoramidite ligands (e.g., TF-BiphamPhos), the complex achieves enantioselective synthesis of α,β-diamino acid esters (ee >95%) .
Trifluoromethylation
Catalyzes trifluoromethylation of cyclopropanols using Togni reagent, yielding β-trifluoromethyl ketones (70–85% yields) .
Dynamic Covalent Chemistry
In aqueous media, [Cu(CH₃CN)₄]BF₄ directs the self-assembly of 2:1 macrocycles from sodium benzaldehyde-2-sulfonate and diamines :
Diamine Structure | Macrocycle Stability |
---|---|
Rigid aromatic | High (ΔG < −30 kJ/mol) |
Flexible aliphatic | Moderate (ΔG ~ −20 kJ/mol) |
Reductive Cross-Coupling
Enables one-pot synthesis of secondary/tertiary amides from aldehydes and amides via:
Comparative Reactivity with Analogues
Property | [Cu(CH₃CN)₄]BF₄ | [Cu(CH₃CN)₄]PF₆ | [Cu(CH₃CN)₄]ClO₄ |
---|---|---|---|
Solubility in MeCN | High | High | Moderate |
Thermal Stability (°C) | 164 (dec.) | 180 (dec.) | 150 (dec.) |
Catalytic Efficiency | +++ | ++ | + |
Aplicaciones Científicas De Investigación
Tetrakis(acetonitrile)copper(I) tetrafluoroborate is widely used in scientific research and industrial applications, including:
Mecanismo De Acción
The acetonitrile ligands in tetrakis(acetonitrile)copper(I) tetrafluoroborate protect the copper(I) ion from oxidation to copper(II). The ligands are rather poorly bound, allowing the complex to form di- and tri-acetonitrilo complexes with other counterions. This property makes the compound a useful source of unbound copper(I) ions .
Comparación Con Compuestos Similares
Structural and Ligand-Based Comparisons
Tetrakis(acetonitrile)silver(I) Tetrafluoroborate ([Ag(CH₃CN)₄]BF₄)
- Structure : Similar cationic framework but with Ag⁺ instead of Cu⁺.
- Reactivity : Silver(I) complexes are less redox-active than copper(I), limiting their utility in radical-mediated reactions (e.g., diazonium salt reductions) .
- Applications : Primarily used in photochemistry, lacking the broad catalytic versatility of copper analogs .
Hypercoordinated Gold Complexes (e.g., [H₃C–C(AuPPh₃)₄]BF₄)
- Structure : Tetraaurio ethanium cations with Au⁺ centers and phosphine ligands .
- Stability : Higher thermal stability (decomposition at 162°C vs. [Cu(CH₃CN)₄]BF₄’s lower stability in aqueous conditions) .
- Applications : Specialized in supramolecular chemistry and gold cluster synthesis, unlike copper analogs used in catalysis .
Counterion Effects
Tetrafluoroborate (BF₄⁻) vs. Hexafluorophosphate (PF₆⁻)
- Reactivity : BF₄⁻ hydrolyzes to release fluoride ions, which enhance electrophilicity in catalytic cycles. PF₆⁻ is inert, leading to a 98% drop in HPLC yield for sulfone formation when substituted .
- Coordination Strength : BF₄⁻ is weakly coordinating, preserving the labile acetonitrile ligands in [Cu(CH₃CN)₄]⁺, whereas PF₆⁻ may induce ligand displacement .
Hydrogen Oxalate (HC₂O₄⁻) in [Cu(CH₃CN)₄]HC₂O₄
- Solubility: Lower solubility in non-polar solvents compared to BF₄⁻ analogs .
Catalytic Performance
Copper(I) vs. Dirhodium(II) Catalysts
- Reaction Divergence :
Comparison with Cobalt(III) Complexes
- Complex Stability : Cobalt(III) complexes (e.g., [Co(P(OⁱPr)₃)₂(BzNC)₄]BF₄) exhibit higher thermal stability but require harsher reaction conditions .
- Catalytic Scope: Limited to hydrogenation and isomerization, unlike copper(I)’s versatility in cross-coupling and radical reactions .
Data Tables
Table 1: Comparative Catalytic Yields
Table 2: Antibacterial Performance of MOFs
MOF Ligand | Inhibition Zone (mm, Light/Dark) | Reference |
---|---|---|
3,5-Dimethyl-1,2,4-triazole | 39.45 / 37.08 | |
2,6-Pyridinedicarboxylic acid | 38.91 / 39.06 | |
Benzene-1,3,5-tricarboxaldehyde | 31.49 / 36.04 |
Actividad Biológica
Tetrakis(acetonitrile)copper(I) tetrafluoroborate, commonly referred to as Cu(MeCN)₄BF₄, is a copper coordination complex that has garnered attention for its diverse biological and catalytic activities. This article explores its biological activity, including its mechanisms of action, potential applications in medicine, and relevant research findings.
- Chemical Formula : C₈H₁₂BCuF₄N₄
- Molar Mass : 314.56 g/mol
- Structure : The compound consists of a copper(I) ion coordinated to four acetonitrile ligands, with tetrafluoroborate as the counterion. It forms colorless crystals and is isomorphous with other copper complexes.
This compound primarily functions as a catalyst in various organic reactions. Its biological activity can be attributed to several key mechanisms:
- Catalytic Activity : It facilitates chemical transformations by lowering activation energy, thus enhancing reaction rates. This property is particularly useful in organic synthesis, including Diels-Alder reactions and the Ullmann reaction .
- Redox Properties : The compound exhibits notable redox activity, allowing it to participate in electron transfer processes. This characteristic is crucial for its applications in catalysis and potential therapeutic uses .
1. Catalysis in Organic Synthesis
This compound is widely used in synthetic chemistry due to its efficiency as a catalyst. It has been employed in:
- Diels-Alder Reactions : It catalyzes the formation of cyclohexenes from diene and dienophile substrates.
- Ullmann Coupling : Facilitates the coupling of aryl halides with nucleophiles .
2. Radiopharmaceuticals
The compound plays a significant role in the production of technetium (99mTc)-sestamibi, a radiopharmaceutical used in nuclear medicine for imaging purposes . Its ability to coordinate with technetium enhances the stability and efficacy of the resulting compounds.
3. Green Chemistry
This compound is also recognized for its application in greener oxidation processes, such as the aerobic oxidation of alcohols to aldehydes under mild conditions . This aligns with sustainable practices in chemical synthesis.
Toxicological Profile
While this compound is valuable in various applications, it also poses certain health risks:
- Toxicity : Exposure can lead to chemical burns and gastrointestinal damage upon ingestion. Symptoms may include metallic taste, nausea, and vomiting .
- Environmental Impact : As with many heavy metal complexes, care must be taken regarding disposal and environmental contamination.
Case Studies and Research Findings
Several studies have highlighted the biological activity and potential applications of this compound:
- A study investigated its role in catalyzing the CuAAC (Copper-catalyzed Azide-Alkyne Cycloaddition) reaction under aerobic conditions, demonstrating its effectiveness compared to other catalysts .
- Another research highlighted its use in synthesizing functionalized copper complexes that exhibit enhanced catalytic properties for various organic transformations .
Summary Table of Biological Activities
Q & A
Basic Research Questions
Q. What are the key considerations for synthesizing tetrakis(acetonitrile)copper(I) tetrafluoroborate ([Cu(CH₃CN)₄]BF₄) with high purity?
- Methodological Answer : Synthesis typically involves reacting copper(I) salts (e.g., CuCl) with tetrafluoroboric acid (HBF₄) in anhydrous acetonitrile under inert atmospheres. Key steps include:
- Strict exclusion of oxygen and moisture to prevent oxidation to Cu(II) species .
- Use of degassed solvents and Schlenk-line techniques for ligand exchange .
- Recrystallization from acetonitrile/diethyl ether mixtures to isolate pure crystals .
- Purity Validation : Characterize via X-ray diffraction (XRD) for structural confirmation and elemental analysis to verify stoichiometry.
Q. How should [Cu(CH₃CN)₄]BF₄ be handled to ensure safety and stability in laboratory settings?
- Safety Protocols :
- Use nitrile gloves, safety goggles, and lab coats to avoid skin/eye contact (classified as skin corrosive/irritant) .
- Store under inert gas (argon) at –20°C to prevent ligand dissociation and moisture absorption .
- Decomposition Risks : Avoid exposure to oxidizing agents (e.g., O₂, HNO₃) and strong bases, which trigger ligand loss or BF₄⁻ hydrolysis .
Q. What are the primary applications of [Cu(CH₃CN)₄]BF₄ in coordination chemistry?
- Coordination Template : Serves as a labile Cu(I) source for constructing supramolecular assemblies (e.g., Cu₁₂L₈ cages) via ligand substitution .
- Catalytic Precursor : Activates alkyne-azide cycloaddition (CuAAC) reactions by generating reactive Cu(I)-acetylide intermediates .
Advanced Research Questions
Q. How do solvent polarity and counterion choice influence the reactivity of [Cu(CH₃CN)₄]⁺ in catalytic cycles?
- Solvent Effects : Polar aprotic solvents (e.g., acetonitrile) stabilize the Cu(I) center by preventing ligand displacement, enhancing catalytic turnover in redox processes .
- Counterion Impact : BF₄⁻ is weakly coordinating, allowing facile ligand exchange, whereas PF₆⁻ or SbF₆⁻ may slow kinetics due to stronger ion pairing .
- Table 1 : Solvent/Counterion Optimization
Solvent | Counterion | Reaction Rate (k, s⁻¹) | Application Example |
---|---|---|---|
Acetonitrile | BF₄⁻ | 0.45 | CuAAC catalysis |
Acetone | PF₆⁻ | 0.12 | Electrochemical CO₂ reduction |
Q. What mechanistic insights explain contradictions in Cu(I) speciation during CuAAC reactions?
- Contradiction : While [Cu(CH₃CN)₄]⁺ is a common precatalyst, some studies report dinuclear Cu(I)-ligand complexes as active species .
- Resolution :
- In-situ EXAFS/EPR : Reveals dynamic equilibria between mononuclear [Cu(CH₃CN)₄]⁺ and dinuclear [Cu₂(μ-L)₂]²⁺ species, depending on ligand denticity .
- Kinetic Profiling : Rate acceleration with TBTA ligands supports dinuclear intermediates stabilizing transition states .
Q. What advanced techniques are recommended for characterizing [Cu(CH₃CN)₄]BF₄-derived complexes?
- Structural Analysis :
- Single-crystal XRD for bond-length determination (e.g., Cu–N distances ~2.0 Å in tetrahedral geometry) .
- FT-IR to monitor acetonitrile ligand vibrations (ν(C≡N) ~2250 cm⁻¹) .
Q. How can researchers address challenges in synthesizing air-sensitive Cu(I) intermediates using [Cu(CH₃CN)₄]BF₄?
- Advanced Techniques :
- Glovebox Synthesis : Ensures O₂-free environments for ligand substitution (e.g., with phosphines or N-heterocyclic carbenes) .
- Stoichiometric Trapping : Use chelating ligands (e.g., phenanthroline) to stabilize transient Cu(I) species for isolation .
Q. Data Contradiction Analysis
Q. Why do studies report conflicting catalytic efficiencies for [Cu(CH₃CN)₄]BF₄ in CO₂ electroreduction?
- Key Factors :
- Electrode Composition : Carbon-supported Cu₄BHT (derived from [Cu(CH₃CN)₄]BF₄) shows higher acetate selectivity vs. Cu foil due to charge-ordered Cu⁺/Cu²⁺ sites .
- Electrolyte pH : Acidic conditions favor C₂ product formation, while neutral pH promotes CH₄ .
- Resolution : Standardize testing protocols (e.g., H-cell vs. flow reactors) to isolate material vs. operational variables.
Q. Methodological Recommendations
Q. What strategies optimize ligand substitution in [Cu(CH₃CN)₄]BF₄ for tailored catalysis?
- Stepwise Approach :
Ligand Screening : Test bidentate vs. tridentate ligands for kinetic stability (e.g., TBTA vs. phenanthroline) .
Solvent Selection : Use low-donor-number solvents (e.g., CH₂Cl₂) to accelerate ligand exchange .
- Case Study : Substitution with 2,9-dimethyl-1,10-phenanthroline yields air-stable Cu(I) complexes for asymmetric catalysis .
Propiedades
IUPAC Name |
acetonitrile;copper(1+);tetrafluoroborate | |
---|---|---|
Source | PubChem | |
URL | https://pubchem.ncbi.nlm.nih.gov | |
Description | Data deposited in or computed by PubChem | |
InChI |
InChI=1S/4C2H3N.BF4.Cu/c4*1-2-3;2-1(3,4)5;/h4*1H3;;/q;;;;-1;+1 | |
Source | PubChem | |
URL | https://pubchem.ncbi.nlm.nih.gov | |
Description | Data deposited in or computed by PubChem | |
InChI Key |
YZGSKMIIVMCEFE-UHFFFAOYSA-N | |
Source | PubChem | |
URL | https://pubchem.ncbi.nlm.nih.gov | |
Description | Data deposited in or computed by PubChem | |
Canonical SMILES |
[B-](F)(F)(F)F.CC#N.CC#N.CC#N.CC#N.[Cu+] | |
Source | PubChem | |
URL | https://pubchem.ncbi.nlm.nih.gov | |
Description | Data deposited in or computed by PubChem | |
Molecular Formula |
C8H12BCuF4N4 | |
Source | PubChem | |
URL | https://pubchem.ncbi.nlm.nih.gov | |
Description | Data deposited in or computed by PubChem | |
DSSTOX Substance ID |
DTXSID80451051 | |
Record name | Tetrakis(acetonitrile)copper(I) tetrafluoroborate | |
Source | EPA DSSTox | |
URL | https://comptox.epa.gov/dashboard/DTXSID80451051 | |
Description | DSSTox provides a high quality public chemistry resource for supporting improved predictive toxicology. | |
Molecular Weight |
314.56 g/mol | |
Source | PubChem | |
URL | https://pubchem.ncbi.nlm.nih.gov | |
Description | Data deposited in or computed by PubChem | |
CAS No. |
15418-29-8 | |
Record name | Tetrakis(acetonitrile)copper(I) tetrafluoroborate | |
Source | EPA DSSTox | |
URL | https://comptox.epa.gov/dashboard/DTXSID80451051 | |
Description | DSSTox provides a high quality public chemistry resource for supporting improved predictive toxicology. | |
Record name | Tetrakis(acetonitrile)copper(I) Tetrafluoroborate | |
Source | European Chemicals Agency (ECHA) | |
URL | https://echa.europa.eu/information-on-chemicals | |
Description | The European Chemicals Agency (ECHA) is an agency of the European Union which is the driving force among regulatory authorities in implementing the EU's groundbreaking chemicals legislation for the benefit of human health and the environment as well as for innovation and competitiveness. | |
Explanation | Use of the information, documents and data from the ECHA website is subject to the terms and conditions of this Legal Notice, and subject to other binding limitations provided for under applicable law, the information, documents and data made available on the ECHA website may be reproduced, distributed and/or used, totally or in part, for non-commercial purposes provided that ECHA is acknowledged as the source: "Source: European Chemicals Agency, http://echa.europa.eu/". Such acknowledgement must be included in each copy of the material. ECHA permits and encourages organisations and individuals to create links to the ECHA website under the following cumulative conditions: Links can only be made to webpages that provide a link to the Legal Notice page. | |
Retrosynthesis Analysis
AI-Powered Synthesis Planning: Our tool employs the Template_relevance Pistachio, Template_relevance Bkms_metabolic, Template_relevance Pistachio_ringbreaker, Template_relevance Reaxys, Template_relevance Reaxys_biocatalysis model, leveraging a vast database of chemical reactions to predict feasible synthetic routes.
One-Step Synthesis Focus: Specifically designed for one-step synthesis, it provides concise and direct routes for your target compounds, streamlining the synthesis process.
Accurate Predictions: Utilizing the extensive PISTACHIO, BKMS_METABOLIC, PISTACHIO_RINGBREAKER, REAXYS, REAXYS_BIOCATALYSIS database, our tool offers high-accuracy predictions, reflecting the latest in chemical research and data.
Strategy Settings
Precursor scoring | Relevance Heuristic |
---|---|
Min. plausibility | 0.01 |
Model | Template_relevance |
Template Set | Pistachio/Bkms_metabolic/Pistachio_ringbreaker/Reaxys/Reaxys_biocatalysis |
Top-N result to add to graph | 6 |
Feasible Synthetic Routes
Descargo de responsabilidad e información sobre productos de investigación in vitro
Tenga en cuenta que todos los artículos e información de productos presentados en BenchChem están destinados únicamente con fines informativos. Los productos disponibles para la compra en BenchChem están diseñados específicamente para estudios in vitro, que se realizan fuera de organismos vivos. Los estudios in vitro, derivados del término latino "in vidrio", involucran experimentos realizados en entornos de laboratorio controlados utilizando células o tejidos. Es importante tener en cuenta que estos productos no se clasifican como medicamentos y no han recibido la aprobación de la FDA para la prevención, tratamiento o cura de ninguna condición médica, dolencia o enfermedad. Debemos enfatizar que cualquier forma de introducción corporal de estos productos en humanos o animales está estrictamente prohibida por ley. Es esencial adherirse a estas pautas para garantizar el cumplimiento de los estándares legales y éticos en la investigación y experimentación.