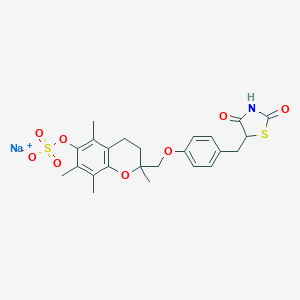
Troglitazone Sulfate Sodium
Descripción general
Descripción
Troglitazone Sulfate Sodium is a derivative of Troglitazone, a thiazolidinedione class compound that was initially developed as an antidiabetic and anti-inflammatory drug. It was primarily used for the treatment of Type II diabetes mellitus by improving insulin sensitivity in muscle and adipose tissue and inhibiting hepatic gluconeogenesis . due to its association with severe hepatotoxicity, Troglitazone was withdrawn from the market in the early 2000s .
Métodos De Preparación
Synthetic Routes and Reaction Conditions: The synthesis of Troglitazone involves the formation of a thiazolidinedione ring structure, which is a key feature of its chemical composition. The process typically includes the following steps:
Formation of the Thiazolidinedione Ring: This involves the reaction of a suitable diketone with thiourea under acidic conditions to form the thiazolidinedione core.
Attachment of the Benzyl Group: The thiazolidinedione core is then reacted with a benzyl halide in the presence of a base to attach the benzyl group.
Introduction of the Chroman Ring:
Industrial Production Methods: Industrial production of Troglitazone Sulfate Sodium follows similar synthetic routes but on a larger scale. The process involves optimization of reaction conditions to ensure high yield and purity of the final product. Key considerations include temperature control, reaction time, and the use of appropriate solvents and catalysts .
Types of Reactions:
Reduction: Reduction reactions are less common but can occur under specific conditions.
Substitution: Troglitazone can undergo substitution reactions, particularly in the presence of strong nucleophiles.
Common Reagents and Conditions:
Oxidation: Common oxidizing agents include cytochrome P450 enzymes in the liver.
Reduction: Reducing agents such as sodium borohydride can be used under controlled conditions.
Substitution: Strong nucleophiles such as alkoxides or amines can facilitate substitution reactions.
Major Products:
Oxidation Products: Quinone and epoxide species.
Reduction Products: Reduced forms of Troglitazone.
Substitution Products: Various substituted derivatives depending on the nucleophile used.
Aplicaciones Científicas De Investigación
Diabetes Management
Troglitazone was initially approved for the management of type 2 diabetes. Clinical studies demonstrated its efficacy in improving metabolic control:
- In a study involving NIDDM patients, Troglitazone significantly lowered fasting plasma insulin concentrations and improved insulin sensitivity as measured by the homeostasis model assessment (HOMA) after 12 weeks of treatment .
- It also positively influenced lipid profiles, increasing HDL cholesterol levels while managing triglycerides .
Neuroprotection
Recent studies have indicated that Troglitazone may have neuroprotective properties. Research showed that Troglitazone could protect neuronal cells from prion protein-induced toxicity. In vitro experiments revealed that pretreatment with Troglitazone significantly increased cell viability in the presence of toxic prion proteins, suggesting a potential application in neurodegenerative diseases .
Safety Concerns and Liver Toxicity
Despite its therapeutic benefits, Troglitazone's clinical use was marred by significant safety concerns, particularly regarding liver toxicity:
- A comprehensive analysis of liver failure cases associated with Troglitazone use revealed that the incidence of acute liver failure was notably high, with many cases progressing rapidly to irreversible injury within one month of treatment . The estimated number needed to harm was between 600 to 1500 patients over 26 months .
- Monitoring liver function tests was deemed insufficient for preventing severe outcomes, indicating a need for caution in its use .
Case Study: Liver Failure
A notable case study evaluated multiple instances of liver failure linked to Troglitazone usage. Out of 94 reported cases, the majority were acute and predominantly affected women. The study emphasized the rapid progression from normal liver function to acute failure, raising concerns about the safety profile of Troglitazone in clinical practice .
Comparative Data Table
Mecanismo De Acción
Troglitazone Sulfate Sodium exerts its effects primarily through the activation of peroxisome proliferator-activated receptors (PPARs), specifically PPARα and PPARγ . These nuclear receptors regulate the transcription of genes involved in glucose and lipid metabolism. By binding to these receptors, Troglitazone enhances insulin sensitivity, decreases hepatic glucose output, and increases insulin-dependent glucose disposal in skeletal muscle . Additionally, Troglitazone has been shown to reduce inflammation by decreasing nuclear factor kappa-B (NF-κB) activity and increasing its inhibitor (IκB) .
Comparación Con Compuestos Similares
Pioglitazone: Another thiazolidinedione with a similar mechanism of action but a better safety profile.
Rosiglitazone: Similar to Pioglitazone, it activates PPARγ but has been associated with cardiovascular risks.
Uniqueness of Troglitazone Sulfate Sodium:
Unique Structure: The presence of a chroman ring structure, which is not found in other thiazolidinediones.
Vitamin E-like Activity: The α-tocopherol moiety in Troglitazone provides additional antioxidant properties.
Hepatotoxicity: Unlike its counterparts, Troglitazone’s use was limited due to its severe hepatotoxicity.
This compound remains a compound of interest in scientific research despite its withdrawal from clinical use. Its unique chemical structure and mechanism of action continue to provide valuable insights into the development of safer and more effective therapeutic agents.
Propiedades
IUPAC Name |
sodium;[2-[[4-[(2,4-dioxo-1,3-thiazolidin-5-yl)methyl]phenoxy]methyl]-2,5,7,8-tetramethyl-3,4-dihydrochromen-6-yl] sulfate | |
---|---|---|
Source | PubChem | |
URL | https://pubchem.ncbi.nlm.nih.gov | |
Description | Data deposited in or computed by PubChem | |
InChI |
InChI=1S/C24H27NO8S2.Na/c1-13-14(2)21-18(15(3)20(13)33-35(28,29)30)9-10-24(4,32-21)12-31-17-7-5-16(6-8-17)11-19-22(26)25-23(27)34-19;/h5-8,19H,9-12H2,1-4H3,(H,25,26,27)(H,28,29,30);/q;+1/p-1 | |
Source | PubChem | |
URL | https://pubchem.ncbi.nlm.nih.gov | |
Description | Data deposited in or computed by PubChem | |
InChI Key |
QDIKTIPHNIELCY-UHFFFAOYSA-M | |
Source | PubChem | |
URL | https://pubchem.ncbi.nlm.nih.gov | |
Description | Data deposited in or computed by PubChem | |
Canonical SMILES |
CC1=C(C(=C(C2=C1OC(CC2)(C)COC3=CC=C(C=C3)CC4C(=O)NC(=O)S4)C)OS(=O)(=O)[O-])C.[Na+] | |
Source | PubChem | |
URL | https://pubchem.ncbi.nlm.nih.gov | |
Description | Data deposited in or computed by PubChem | |
Molecular Formula |
C24H26NNaO8S2 | |
Source | PubChem | |
URL | https://pubchem.ncbi.nlm.nih.gov | |
Description | Data deposited in or computed by PubChem | |
Molecular Weight |
543.6 g/mol | |
Source | PubChem | |
URL | https://pubchem.ncbi.nlm.nih.gov | |
Description | Data deposited in or computed by PubChem | |
Retrosynthesis Analysis
AI-Powered Synthesis Planning: Our tool employs the Template_relevance Pistachio, Template_relevance Bkms_metabolic, Template_relevance Pistachio_ringbreaker, Template_relevance Reaxys, Template_relevance Reaxys_biocatalysis model, leveraging a vast database of chemical reactions to predict feasible synthetic routes.
One-Step Synthesis Focus: Specifically designed for one-step synthesis, it provides concise and direct routes for your target compounds, streamlining the synthesis process.
Accurate Predictions: Utilizing the extensive PISTACHIO, BKMS_METABOLIC, PISTACHIO_RINGBREAKER, REAXYS, REAXYS_BIOCATALYSIS database, our tool offers high-accuracy predictions, reflecting the latest in chemical research and data.
Strategy Settings
Precursor scoring | Relevance Heuristic |
---|---|
Min. plausibility | 0.01 |
Model | Template_relevance |
Template Set | Pistachio/Bkms_metabolic/Pistachio_ringbreaker/Reaxys/Reaxys_biocatalysis |
Top-N result to add to graph | 6 |
Feasible Synthetic Routes
Descargo de responsabilidad e información sobre productos de investigación in vitro
Tenga en cuenta que todos los artículos e información de productos presentados en BenchChem están destinados únicamente con fines informativos. Los productos disponibles para la compra en BenchChem están diseñados específicamente para estudios in vitro, que se realizan fuera de organismos vivos. Los estudios in vitro, derivados del término latino "in vidrio", involucran experimentos realizados en entornos de laboratorio controlados utilizando células o tejidos. Es importante tener en cuenta que estos productos no se clasifican como medicamentos y no han recibido la aprobación de la FDA para la prevención, tratamiento o cura de ninguna condición médica, dolencia o enfermedad. Debemos enfatizar que cualquier forma de introducción corporal de estos productos en humanos o animales está estrictamente prohibida por ley. Es esencial adherirse a estas pautas para garantizar el cumplimiento de los estándares legales y éticos en la investigación y experimentación.