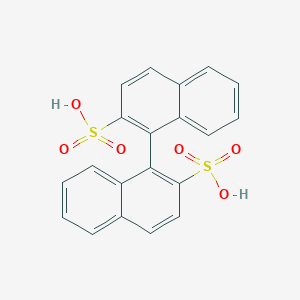
1-(2-Sulfo-1-naphthyl)naphthalene-2-sulfonic acid
- Haga clic en CONSULTA RÁPIDA para recibir una cotización de nuestro equipo de expertos.
- Con productos de calidad a un precio COMPETITIVO, puede centrarse más en su investigación.
Descripción general
Descripción
Naphthalene-1-sulfonic acid and naphthalene-2-sulfonic acid are organic compounds with the formula C10H7SO3H . They are colorless, water-soluble solids often available as hydrates . They are two mono sulfonic acids of naphthalene .
Synthesis Analysis
Naphthalene-2-sulfonic acid is prepared by sulfonation of naphthalene using 98% sulfuric acid as a sulfonating agent at 160-166℃ . During the sulfonation process, a small amount of α-naphthalenesulfonic acid by-product is generated .Molecular Structure Analysis
The molecular formula for these compounds is C10H8O3S . The molecular weight is 208.234 Da . The 3D structure may be viewed using Java or Javascript .Chemical Reactions Analysis
Naphthalene-1-sulfonic acid undergoes many reactions. Upon heating with dilute aqueous acid, it reverts to naphthalene. Fusion with sodium hydroxide followed by acidification gives 1-naphthol . Naphthalene-2-sulfonic acid also undergoes many reactions. Fusion with sodium hydroxide followed by acidification gives 2-naphthol .Physical And Chemical Properties Analysis
These compounds are colorless, water-soluble solids . They have good solubility in water . The melting point of naphthalene-2-sulfonic acid is 124 °C (255 °F; 397 K) for the monohydrate , and for naphthalene-1-sulfonic acid, it is 139–140 °C (282–284 °F; 412–413 K) .Safety and Hazards
Propiedades
IUPAC Name |
1-(2-sulfonaphthalen-1-yl)naphthalene-2-sulfonic acid |
Source
|
---|---|---|
Source | PubChem | |
URL | https://pubchem.ncbi.nlm.nih.gov | |
Description | Data deposited in or computed by PubChem | |
InChI |
InChI=1S/C20H14O6S2/c21-27(22,23)17-11-9-13-5-1-3-7-15(13)19(17)20-16-8-4-2-6-14(16)10-12-18(20)28(24,25)26/h1-12H,(H,21,22,23)(H,24,25,26) |
Source
|
Source | PubChem | |
URL | https://pubchem.ncbi.nlm.nih.gov | |
Description | Data deposited in or computed by PubChem | |
InChI Key |
ZSWODTYSMOCDKE-UHFFFAOYSA-N |
Source
|
Source | PubChem | |
URL | https://pubchem.ncbi.nlm.nih.gov | |
Description | Data deposited in or computed by PubChem | |
Canonical SMILES |
C1=CC=C2C(=C1)C=CC(=C2C3=C(C=CC4=CC=CC=C43)S(=O)(=O)O)S(=O)(=O)O |
Source
|
Source | PubChem | |
URL | https://pubchem.ncbi.nlm.nih.gov | |
Description | Data deposited in or computed by PubChem | |
Molecular Formula |
C20H14O6S2 |
Source
|
Source | PubChem | |
URL | https://pubchem.ncbi.nlm.nih.gov | |
Description | Data deposited in or computed by PubChem | |
Molecular Weight |
414.5 g/mol |
Source
|
Source | PubChem | |
URL | https://pubchem.ncbi.nlm.nih.gov | |
Description | Data deposited in or computed by PubChem | |
Descargo de responsabilidad e información sobre productos de investigación in vitro
Tenga en cuenta que todos los artículos e información de productos presentados en BenchChem están destinados únicamente con fines informativos. Los productos disponibles para la compra en BenchChem están diseñados específicamente para estudios in vitro, que se realizan fuera de organismos vivos. Los estudios in vitro, derivados del término latino "in vidrio", involucran experimentos realizados en entornos de laboratorio controlados utilizando células o tejidos. Es importante tener en cuenta que estos productos no se clasifican como medicamentos y no han recibido la aprobación de la FDA para la prevención, tratamiento o cura de ninguna condición médica, dolencia o enfermedad. Debemos enfatizar que cualquier forma de introducción corporal de estos productos en humanos o animales está estrictamente prohibida por ley. Es esencial adherirse a estas pautas para garantizar el cumplimiento de los estándares legales y éticos en la investigación y experimentación.