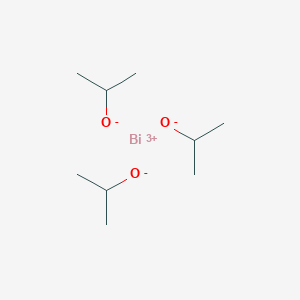
Bismuth(III) isopropoxide
Descripción general
Descripción
Bismuth(III) isopropoxide (CAS: 15049-67-9) is a metal alkoxide with the molecular formula C₉H₂₁BiO₃ and a molecular weight of 386.24 g/mol. This compound is characterized by its sensitivity to moisture, reacting with water to form bismuth oxides, and its solubility in organic solvents such as ethanol and toluene . Its steric and electronic properties enable selective reactivity, making it valuable in coordination chemistry and catalysis. Applications span organic synthesis, sol-gel processing, and the preparation of bismuth-containing nanomaterials. Notably, its low toxicity compared to other heavy metal alkoxides aligns with growing demands for environmentally benign reagents .
Métodos De Preparación
Direct Synthesis from Bismuth Metal and Isopropanol
The direct reaction between bismuth metal and isopropanol represents a foundational method for synthesizing bismuth(III) isopropoxide. This approach leverages the redox activity of bismuth in alcoholic media, often facilitated by catalytic agents.
Reaction Mechanism and Conditions
Bismuth metal reacts with isopropanol in the presence of a mercury(II) chloride (HgCl₂) catalyst under anhydrous conditions. The reaction proceeds via oxidative dissolution of bismuth, yielding this compound and hydrogen gas:
3\text{CHOHCH}3 \xrightarrow{\text{HgCl}2} 2 \, \text{Bi(OCH(CH}3\text{)}2\text{)}3 + 3 \, \text{H}_2 \uparrow
Key parameters include:
-
Temperature : 80–100°C to accelerate reaction kinetics.
-
Catalyst loading : 0.5–1.0 wt% HgCl₂ relative to bismuth.
-
Solvent : Dry isopropanol to prevent hydrolysis.
Yield and Purity Optimization
Table 1 summarizes the impact of reaction time and temperature on yield. Prolonged heating (>24 hours) at 90°C achieves yields up to 78%, but excessive temperatures (>110°C) promote side reactions, reducing purity .
Table 1: Effect of Reaction Conditions on Direct Synthesis
Temperature (°C) | Time (hours) | Yield (%) | Purity (Bi wt%) |
---|---|---|---|
80 | 18 | 62 | 94.5 |
90 | 24 | 78 | 98.2 |
100 | 24 | 71 | 92.8 |
Metathesis Reactions with Bismuth Halides
Metathesis between bismuth halides (e.g., BiCl₃) and alkali metal isopropoxides (e.g., NaOCH(CH₃)₂) is a widely adopted method for large-scale production.
Stoichiometric Considerations
The reaction follows:
3 + 3 \, \text{NaOCH(CH}3\text{)}2 \rightarrow \text{Bi(OCH(CH}3\text{)}2\text{)}3 + 3 \, \text{NaCl}
Key considerations include:
-
Solvent choice : Toluene or hexane to solubilize reactants and precipitate NaCl.
-
Molar ratio : A 1:3.2 BiCl₃-to-alkoxide ratio ensures complete conversion.
Industrial-Scale Refinement
Industrial protocols often employ continuous distillation to remove NaCl and excess alkoxide. Pilot studies report 85–90% yields with residual sodium <50 ppm .
Transesterification of Lower Bismuth Alkoxides
Transesterification allows the conversion of more accessible bismuth alkoxides (e.g., bismuth ethoxide) into the isopropoxide derivative.
Reaction Dynamics
Using excess isopropanol, the equilibrium shifts toward this compound:
2\text{CH}3\text{)}3 + 3 \, \text{CH}3\text{CHOHCH}3 \rightleftharpoons \text{Bi(OCH(CH}3\text{)}2\text{)}3 + 3 \, \text{CH}3\text{CH}2\text{OH}
-
Catalysts : Titanium tetraisopropoxide (Ti(OCH(CH₃)₂)₄) at 0.1–0.5 mol% enhances reaction rates.
-
Azeotropic removal : Ethanol is distilled off to drive the reaction to completion.
Scalability and Economic Viability
This method is cost-effective for facilities already producing bismuth ethoxide. Batch processes achieve 92% conversion in 8–12 hours, with product purity >99% after vacuum distillation .
Solvothermal Synthesis for Nanostructured Products
Emerging solvothermal techniques enable the production of nanostructured this compound with tailored morphologies.
Methodology
A mixture of bismuth nitrate and isopropanol is heated in an autoclave at 150–200°C for 6–12 hours. The high-pressure environment promotes the formation of colloidal bismuth isopropoxide particles.
Structural Characterization
Transmission electron microscopy (TEM) reveals spherical nanoparticles (20–50 nm) with narrow size distributions. X-ray diffraction (XRD) confirms crystallinity matching the orthorhombic phase (JCPDS 00-043-0449) .
Quality Control and Analytical Techniques
Purity Assessment
-
Elemental analysis : Inductively coupled plasma mass spectrometry (ICP-MS) quantifies bismuth content (expected: 45.2 wt%).
-
FT-IR spectroscopy : Peaks at 980 cm⁻¹ (Bi–O stretching) and 1120 cm⁻¹ (C–O bending) confirm structural integrity.
Análisis De Reacciones Químicas
Hydrolysis and Solvolysis
Bismuth(III) isopropoxide undergoes rapid hydrolysis in aqueous or protic environments due to the strong Lewis acidity of Bi³⁺. The reaction proceeds via ligand exchange:
Key Observations :
-
Hydrolysis generates bismuth hydroxide (Bi(OH)₃) and isopropanol .
-
In acidic conditions, Bi(OH)₃ dissolves to form Bi³⁺ ions :
-
In the presence of chloride ions, hydrolysis yields bismuth oxychloride (BiOCl) :
Conditions :
Reaction Medium | Product | Notes |
---|---|---|
Neutral water | Bi(OH)₃ | White precipitate |
Acidic solution | Bi³⁺ | Soluble species |
HCl-containing | BiOCl | Crystalline solid |
Oxidation Reactions
This compound acts as a precursor to bismuth oxides under oxidative conditions. Thermal decomposition or reaction with oxidizing agents yields Bi₂O₃:
Key Findings :
-
Ignition of Bi(OH)₃ (derived from hydrolysis) produces Bi₂O₃ .
-
Bi₂O₃ reacts with CO₂ to form bismuth subcarbonate, but this is inhibited in acidic environments .
Oxidizing Agents :
Agent | Conditions | Product |
---|---|---|
O₂ | Thermal (>400°C) | Bi₂O₃ |
H₂O₂ | Aqueous, acidic | Bi₂O₃ |
Reduction Reactions
Reduction of Bi(OiPr)₃ yields metallic bismuth, typically under hydrogen or hydrazine atmospheres:
Experimental Data :
-
Reduction proceeds efficiently at 200–300°C with H₂ gas.
Substitution Reactions
The isopropoxide ligands are readily displaced by stronger nucleophiles, enabling synthesis of diverse bismuth complexes:
Alcohol Exchange
Examples :
-
Ethanol: Forms Bi(OEt)₃.
-
Phenol: Forms Bi(OPh)₃ (requires acid catalysis).
Reaction with Halides
Halide Compatibility :
Halide (X⁻) | Product | Stability |
---|---|---|
Cl⁻ | BiCl₃ | Hygroscopic, soluble in organic solvents |
Br⁻ | BiBr₃ | Red crystalline solid |
Complexation with Organic Ligands
This compound forms stable complexes with multidentate ligands (e.g., carboxylates, Schiff bases):
Case Study :
-
Reaction with fumaric acid produces bismuth oxofumarate, (BiO)₂C₄H₂O₄, via solid-solution interaction .
Mechanistic Insights
Aplicaciones Científicas De Investigación
Catalytic Applications
Bismuth(III) isopropoxide is recognized for its catalytic properties in organic reactions. It serves as a catalyst in several processes, including:
- Transesterification Reactions : It facilitates the conversion of triglycerides into biodiesel and glycerol.
- Polymerization : Used in the synthesis of polymers, it enhances reaction rates and yields.
Case Study: Polymer Synthesis
Research has demonstrated that this compound can effectively catalyze the polymerization of lactides to produce polylactic acid (PLA), a biodegradable polymer. The use of this catalyst results in higher molecular weights and improved thermal properties compared to traditional metal catalysts.
Precursor for Advanced Materials
This compound acts as a precursor for synthesizing various bismuth-based materials with unique properties:
- Superconductors : It is utilized in creating bismuth-based cuprate superconductors, which exhibit high critical temperatures suitable for energy applications.
- Thermoelectric Materials : The compound is pivotal in synthesizing bismuth telluride (), known for its efficiency in converting heat to electricity.
Data Table: Properties of Bismuth-Based Materials
Material | Application | Key Feature |
---|---|---|
Bismuth Telluride | Thermoelectric devices | High thermoelectric efficiency |
Bismuth Cuprate | Superconductors | High critical temperature |
Bismuth Vanadate Pigments | Paints and ceramics | Bright colors and stability |
Photocatalytic Applications
Recent studies have explored the photocatalytic potential of bismuth compounds, including those derived from this compound. These materials are effective in degrading pollutants under UV light.
Case Study: Photocatalysis
A composite of bismuth oxide supported on kaolinite showed enhanced photocatalytic activity for the degradation of organic dyes. The incorporation of this compound improved the surface area and charge transport properties, leading to superior performance .
Biomedical Applications
Bismuth compounds have been investigated for their biological activities, particularly against pathogens such as Helicobacter pylori. This compound can be part of formulations aimed at treating gastrointestinal ailments.
Antimicrobial Activity
Studies indicate that bismuth complexes exhibit significant antibacterial effects against various strains, including MRSA and VRE. The mechanism involves disrupting bacterial metabolic pathways and inhibiting virulence factors .
Mecanismo De Acción
The mechanism of action of Bismuth(III) isopropoxide involves its ability to form complexes with various substrates. In biological systems, it can interact with proteins and enzymes, leading to inhibition or activation of their functions. For example, it has been shown to inhibit the activity of certain enzymes by binding to their active sites.
Comparación Con Compuestos Similares
Comparison with Other Bismuth(III) Compounds
Bismuth(III) Nitrate (Bi(NO₃)₃·5H₂O)
- Molecular Weight : 485.07 g/mol (pentahydrate).
- Reactivity : Soluble in acidic aqueous solutions but hydrolyzes in neutral water to form oxynitrates.
- Applications : Widely used as a precursor for bismuth oxide thin films and perovskite materials (e.g., Bi₄Ti₃O₁₂) in sol-gel synthesis .
- Key Difference : Unlike bismuth(III) isopropoxide, bismuth nitrate is hygroscopic and requires acidic stabilization to prevent hydrolysis.
Bismuth(III) Iodide (BiI₃)
- Molecular Weight : 589.69 g/mol.
- Reactivity : Stable in dry air but decomposes in humid conditions. Insoluble in water.
- Applications : Semiconductor applications due to its layered crystal structure.
- Key Difference : Bismuth iodide lacks the organic ligand system of this compound, limiting its utility in homogeneous catalysis .
Bismuth(III) Oxide (Bi₂O₃)
- Molecular Weight : 465.96 g/mol.
- Reactivity : Insoluble in water but reacts at high temperatures (>600°C) to form suboxides.
- Applications : High-purity ceramics, gas sensors, and photocatalytic materials.
Table 1: Comparative Properties of Bismuth(III) Compounds
Comparison with Other Metal Alkoxides
Titanium Isopropoxide (Ti(OCH(CH₃)₂)₄)
- Molecular Weight : 284.22 g/mol.
- Reactivity: Highly reactive toward hydrolysis, forming TiO₂ nanoparticles.
- Applications: Sol-gel synthesis of titanates, dielectric materials, and nanocomposites.
- Key Difference : Titanium isopropoxide exhibits faster hydrolysis kinetics than this compound, requiring stabilizers like acetylacetone in synthesis .
Zirconium Isopropoxide (Zr(OCH(CH₃)₂)₄)
- Molecular Weight : 327.59 g/mol.
- Reactivity : Similar hydrolysis behavior to titanium isopropoxide, forming ZrO₂.
- Applications : Dopant in photocatalytic materials (e.g., W-/Zr-doped BiVO₄).
- Key Difference : Zirconium alkoxides are more thermally stable but less catalytically versatile than this compound in organic transformations .
Table 2: this compound vs. Transition Metal Alkoxides
Actividad Biológica
Bismuth(III) isopropoxide is an organometallic compound that has garnered attention for its diverse biological activities, particularly in the fields of antimicrobial and anticancer research. This article provides a comprehensive overview of its biological activity, including synthesis, mechanisms of action, and relevant case studies.
This compound, with the formula , is synthesized through various methods, including the reaction of bismuth(III) chloride with isopropanol. The compound appears as a flammable solid and is known for its solubility in organic solvents, which enhances its reactivity in chemical processes.
Antimicrobial Properties
Bismuth compounds, including this compound, have demonstrated significant antimicrobial activity, particularly against Helicobacter pylori. Research indicates that bismuth inhibits the growth of H. pylori by downregulating virulence factors such as CagA and VacA and disrupting metabolic pathways essential for bacterial survival .
Case Study: Inhibition of H. pylori
- Study Findings : Bismuth compounds were effective at low concentrations, with minimum inhibitory concentration (MIC) values reported as low as 8.84 μM against various strains of H. pylori.
- Mechanism : The compound interferes with flagella assembly and antioxidant enzyme activity, leading to bacterial death .
Anticancer Activity
This compound has also been investigated for its anticancer properties. Studies have shown that it can induce apoptosis in cancer cells through various mechanisms, including DNA fragmentation and lipid peroxidation.
Case Study: Cytotoxicity in Cancer Cells
- Cytotoxic Effects : Bismuth complexes have exhibited low toxicity towards normal cells while demonstrating high cytotoxicity against human carcinoma cell lines such as HepG2 (liver cancer) and MCF-7 (breast cancer).
- Mechanisms of Action : The compound promotes apoptosis and inhibits cell cycle progression in cancer cells .
Comparative Biological Activity
The following table summarizes the biological activities of this compound compared to other bismuth compounds:
Compound | Antimicrobial Activity | Anticancer Activity | Toxicity to Normal Cells |
---|---|---|---|
This compound | High | Moderate to High | Low |
Bismuth(III) Nitrate | Moderate | Low | Moderate |
Bismuth(III) Chloride | Low | Low | High |
Mechanisms of Biological Action
The biological activity of this compound can be attributed to several mechanisms:
- Metal Ion Interaction : Bismuth ions can interact with cellular components, leading to disruption of essential biochemical pathways.
- Induction of Oxidative Stress : The compound induces oxidative stress in microbial and cancer cells, resulting in cell death.
- Modulation of Gene Expression : It affects the expression of genes involved in virulence and cell proliferation .
Q & A
Basic Research Questions
Q. What are the optimal methods for synthesizing and characterizing Bismuth(III) isopropoxide?
- Methodology : this compound is typically synthesized via alkoxylation of bismuth salts (e.g., BiCl₃) with isopropanol under anhydrous conditions. Key steps include:
- Reagent drying : Isopropanol must be dried over molecular sieves to avoid hydrolysis .
- Inert atmosphere : Reactions should be conducted under argon/nitrogen to prevent oxidation or moisture interference .
- Characterization : Use NMR (¹H, ¹³C) to confirm ligand coordination, FT-IR for Bi–O bond identification (~500–600 cm⁻¹), and elemental analysis for stoichiometric validation. X-ray crystallography is preferred for structural elucidation but requires high-purity crystals .
Q. How does this compound react with water, and what precautions are necessary during handling?
- Reactivity : It hydrolyzes rapidly in water to form Bi(OH)₃ and isopropanol, releasing heat. This reaction limits its use in aqueous systems .
- Safety protocols : Store under inert gas (argon), use gloveboxes for transfers, and avoid exposure to humid air. Quench residues with dry ethanol before disposal .
Q. What spectroscopic techniques are most reliable for analyzing this compound’s purity?
- Recommended methods :
- FT-IR : Detects residual isopropanol or hydrolysis products (broad O–H stretch at ~3200–3600 cm⁻¹) .
- NMR : Absence of extraneous peaks in ¹H/¹³C spectra confirms ligand integrity .
- TGA : Measures thermal stability; decomposition above 200°C indicates purity .
Advanced Research Questions
Q. How can this compound be utilized as a precursor for bismuth oxide nanomaterials?
- Methodology : Sol-gel processing or chemical vapor deposition (CVD) allows controlled oxide formation. For sol-gel:
- Hydrolyze this compound in a controlled manner (e.g., using acetic acid as a chelating agent) to form Bi₂O₃ nanoparticles .
- Adjust hydrolysis rate via solvent polarity (e.g., toluene vs. THF) to tailor particle size (10–50 nm) .
Q. What role does this compound play in catalytic C–C bond formation, and how does its efficiency compare to other Bi(III) complexes?
- Applications : It catalyzes Mukaiyama aldol and Mannich reactions via Lewis acid activation.
- Efficiency factors :
- Coordination geometry : The isopropoxide ligands enhance solubility in nonpolar solvents, improving substrate access compared to BiCl₃ .
- Turnover frequency (TOF) : Reported TOFs range from 5–50 h⁻¹, depending on substrate steric hindrance.
Q. How can researchers resolve contradictions in reported catalytic yields for this compound-mediated reactions?
- Critical analysis steps :
- Parameter standardization : Compare substrate ratios, solvent purity, and reaction temperature across studies. For example, yields drop by 15–20% if moisture exceeds 50 ppm .
- Byproduct identification : Use GC-MS or HPLC to detect side products (e.g., esterification of isopropanol with acidic substrates) .
- Control experiments : Test catalytic activity of residual Bi(OH)₃ to rule out heterogeneous pathways .
Q. Guidance for Reproducibility
Propiedades
IUPAC Name |
bismuth;propan-2-olate | |
---|---|---|
Source | PubChem | |
URL | https://pubchem.ncbi.nlm.nih.gov | |
Description | Data deposited in or computed by PubChem | |
InChI |
InChI=1S/3C3H7O.Bi/c3*1-3(2)4;/h3*3H,1-2H3;/q3*-1;+3 | |
Source | PubChem | |
URL | https://pubchem.ncbi.nlm.nih.gov | |
Description | Data deposited in or computed by PubChem | |
InChI Key |
KNPRLIQQQKEOJN-UHFFFAOYSA-N | |
Source | PubChem | |
URL | https://pubchem.ncbi.nlm.nih.gov | |
Description | Data deposited in or computed by PubChem | |
Canonical SMILES |
CC(C)[O-].CC(C)[O-].CC(C)[O-].[Bi+3] | |
Source | PubChem | |
URL | https://pubchem.ncbi.nlm.nih.gov | |
Description | Data deposited in or computed by PubChem | |
Molecular Formula |
C9H21BiO3 | |
Source | PubChem | |
URL | https://pubchem.ncbi.nlm.nih.gov | |
Description | Data deposited in or computed by PubChem | |
DSSTOX Substance ID |
DTXSID80627335 | |
Record name | Bismuth(3+) tripropan-2-olate | |
Source | EPA DSSTox | |
URL | https://comptox.epa.gov/dashboard/DTXSID80627335 | |
Description | DSSTox provides a high quality public chemistry resource for supporting improved predictive toxicology. | |
Molecular Weight |
386.24 g/mol | |
Source | PubChem | |
URL | https://pubchem.ncbi.nlm.nih.gov | |
Description | Data deposited in or computed by PubChem | |
CAS No. |
15049-67-9 | |
Record name | Bismuth(3+) tripropan-2-olate | |
Source | EPA DSSTox | |
URL | https://comptox.epa.gov/dashboard/DTXSID80627335 | |
Description | DSSTox provides a high quality public chemistry resource for supporting improved predictive toxicology. | |
Retrosynthesis Analysis
AI-Powered Synthesis Planning: Our tool employs the Template_relevance Pistachio, Template_relevance Bkms_metabolic, Template_relevance Pistachio_ringbreaker, Template_relevance Reaxys, Template_relevance Reaxys_biocatalysis model, leveraging a vast database of chemical reactions to predict feasible synthetic routes.
One-Step Synthesis Focus: Specifically designed for one-step synthesis, it provides concise and direct routes for your target compounds, streamlining the synthesis process.
Accurate Predictions: Utilizing the extensive PISTACHIO, BKMS_METABOLIC, PISTACHIO_RINGBREAKER, REAXYS, REAXYS_BIOCATALYSIS database, our tool offers high-accuracy predictions, reflecting the latest in chemical research and data.
Strategy Settings
Precursor scoring | Relevance Heuristic |
---|---|
Min. plausibility | 0.01 |
Model | Template_relevance |
Template Set | Pistachio/Bkms_metabolic/Pistachio_ringbreaker/Reaxys/Reaxys_biocatalysis |
Top-N result to add to graph | 6 |
Feasible Synthetic Routes
Descargo de responsabilidad e información sobre productos de investigación in vitro
Tenga en cuenta que todos los artículos e información de productos presentados en BenchChem están destinados únicamente con fines informativos. Los productos disponibles para la compra en BenchChem están diseñados específicamente para estudios in vitro, que se realizan fuera de organismos vivos. Los estudios in vitro, derivados del término latino "in vidrio", involucran experimentos realizados en entornos de laboratorio controlados utilizando células o tejidos. Es importante tener en cuenta que estos productos no se clasifican como medicamentos y no han recibido la aprobación de la FDA para la prevención, tratamiento o cura de ninguna condición médica, dolencia o enfermedad. Debemos enfatizar que cualquier forma de introducción corporal de estos productos en humanos o animales está estrictamente prohibida por ley. Es esencial adherirse a estas pautas para garantizar el cumplimiento de los estándares legales y éticos en la investigación y experimentación.