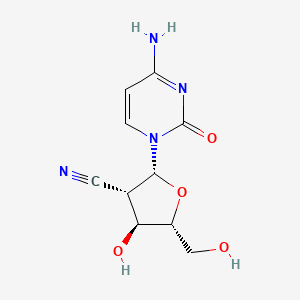
Cndac
Descripción general
Descripción
El ácido etilbutírico, también conocido como ácido 2-etilbutanoico, es un compuesto orgánico con la fórmula molecular C6H12O2. Es un líquido incoloro con un olor característico y es soluble en agua, etanol y éter. El ácido etilbutírico se utiliza comúnmente en la producción de ésteres, que se utilizan en la industria de sabores y fragancias debido a su aroma afrutado.
Aplicaciones Científicas De Investigación
El ácido etilbutírico tiene una amplia gama de aplicaciones en la investigación científica:
Química: Utilizado como reactivo en la síntesis orgánica y como intermedio en la producción de diversos productos químicos.
Biología: Utilizado en el estudio de las vías metabólicas y como estándar en el análisis de ácidos grasos volátiles.
Medicina: Investigado por sus posibles efectos terapéuticos y como agente saborizante en productos farmacéuticos.
Industria: Empleado en la producción de sabores, fragancias y como plastificante en las industrias de polímeros
Mecanismo De Acción
El ácido etilbutírico ejerce sus efectos a través de varios objetivos y vías moleculares. Puede interactuar con enzimas y receptores involucrados en los procesos metabólicos. Por ejemplo, puede actuar como sustrato para enzimas que catalizan la oxidación o reducción de ácidos grasos. Además, sus ésteres pueden unirse a receptores olfativos, contribuyendo a su uso en la industria de sabores y fragancias .
Análisis Bioquímico
Biochemical Properties
Cndac plays a significant role in biochemical reactions by inducing DNA strand breaks . This unique action mechanism distinguishes this compound from other deoxycytidine analogs . This compound interacts with various enzymes and proteins, including SAMHD1 . SAMHD1 mediates intrinsic this compound resistance in leukemia cells . Enzymatic assays and crystallization studies have confirmed this compound triphosphate (this compound-TP) to be a SAMHD1 substrate .
Cellular Effects
This compound has profound effects on various types of cells and cellular processes. It influences cell function by inducing DNA damage and apoptosis
Molecular Mechanism
This compound exerts its effects at the molecular level by inducing DNA strand breaks . This involves binding interactions with biomolecules, enzyme inhibition or activation, and changes in gene expression . For instance, SAMHD1 depletion increases this compound triphosphate (this compound-TP) levels and this compound toxicity .
Metabolic Pathways
This compound is involved in several metabolic pathways. It interacts with enzymes or cofactors, including SAMHD1
Métodos De Preparación
Rutas sintéticas y condiciones de reacción
El ácido etilbutírico se puede sintetizar mediante varios métodos:
Oxidación de 2-etilbutanol: Este método implica la oxidación de 2-etilbutanol utilizando un agente oxidante como permanganato de potasio u óxido de cromo (VI).
Reacción de 3-pentanol con ácido fórmico: En presencia de ácido sulfúrico, el 3-pentanol reacciona con el ácido fórmico para producir ácido etilbutírico.
Descarboxilación de ácido dietilmalónico: Este método implica la descarboxilación del ácido dietilmalónico bajo calor para producir ácido etilbutírico.
Métodos de producción industrial
La producción industrial de ácido etilbutírico normalmente implica la oxidación catalítica de dietilacetaldehído o la descarboxilación de ácido dietilmalónico. Estos métodos son preferidos debido a su eficiencia y escalabilidad .
Análisis De Reacciones Químicas
Tipos de reacciones
El ácido etilbutírico experimenta diversas reacciones químicas, que incluyen:
Oxidación: El ácido etilbutírico se puede oxidar para producir cetonas o aldehídos correspondientes.
Reducción: La reducción de ácido etilbutírico puede producir alcoholes.
Esterificación: El ácido etilbutírico reacciona con alcoholes en presencia de un catalizador ácido para formar ésteres.
Sustitución: Pueden ocurrir reacciones de halogenación, donde los átomos de hidrógeno son reemplazados por átomos de halógeno.
Reactivos y condiciones comunes
Oxidación: Permanganato de potasio, óxido de cromo (VI).
Reducción: Hidruro de litio y aluminio, borohidruro de sodio.
Esterificación: Ácido sulfúrico como catalizador, varios alcoholes.
Sustitución: Halógenos como cloro o bromo.
Productos principales
Oxidación: Cetonas, aldehídos.
Reducción: Alcoholes.
Esterificación: Ésteres.
Sustitución: Derivados halogenados.
Comparación Con Compuestos Similares
El ácido etilbutírico se puede comparar con otros compuestos similares como:
Ácido butírico: Similar en estructura pero carece del grupo etilo, lo que da como resultado diferentes propiedades químicas y aplicaciones.
Ácido isobutírico: Tiene una estructura ramificada, lo que lleva a variaciones en la reactividad y el uso.
Ácido valérico: Contiene un átomo de carbono adicional, lo que afecta sus propiedades físicas y químicas
El ácido etilbutírico es único debido a su estructura específica, que confiere una reactividad química y aplicaciones distintas, particularmente en la industria de sabores y fragancias.
Propiedades
IUPAC Name |
(2R,3S,4S,5R)-2-(4-amino-2-oxopyrimidin-1-yl)-4-hydroxy-5-(hydroxymethyl)oxolane-3-carbonitrile | |
---|---|---|
Source | PubChem | |
URL | https://pubchem.ncbi.nlm.nih.gov | |
Description | Data deposited in or computed by PubChem | |
InChI |
InChI=1S/C10H12N4O4/c11-3-5-8(16)6(4-15)18-9(5)14-2-1-7(12)13-10(14)17/h1-2,5-6,8-9,15-16H,4H2,(H2,12,13,17)/t5-,6+,8-,9+/m0/s1 | |
Source | PubChem | |
URL | https://pubchem.ncbi.nlm.nih.gov | |
Description | Data deposited in or computed by PubChem | |
InChI Key |
DCYBPMFXJCWXNB-JWIUVKOKSA-N | |
Source | PubChem | |
URL | https://pubchem.ncbi.nlm.nih.gov | |
Description | Data deposited in or computed by PubChem | |
Canonical SMILES |
C1=CN(C(=O)N=C1N)C2C(C(C(O2)CO)O)C#N | |
Source | PubChem | |
URL | https://pubchem.ncbi.nlm.nih.gov | |
Description | Data deposited in or computed by PubChem | |
Isomeric SMILES |
C1=CN(C(=O)N=C1N)[C@H]2[C@H]([C@@H]([C@H](O2)CO)O)C#N | |
Source | PubChem | |
URL | https://pubchem.ncbi.nlm.nih.gov | |
Description | Data deposited in or computed by PubChem | |
Molecular Formula |
C10H12N4O4 | |
Source | PubChem | |
URL | https://pubchem.ncbi.nlm.nih.gov | |
Description | Data deposited in or computed by PubChem | |
DSSTOX Substance ID |
DTXSID30159474 | |
Record name | CNDAC | |
Source | EPA DSSTox | |
URL | https://comptox.epa.gov/dashboard/DTXSID30159474 | |
Description | DSSTox provides a high quality public chemistry resource for supporting improved predictive toxicology. | |
Molecular Weight |
252.23 g/mol | |
Source | PubChem | |
URL | https://pubchem.ncbi.nlm.nih.gov | |
Description | Data deposited in or computed by PubChem | |
CAS No. |
135598-68-4 | |
Record name | CNDAC | |
Source | ChemIDplus | |
URL | https://pubchem.ncbi.nlm.nih.gov/substance/?source=chemidplus&sourceid=0135598684 | |
Description | ChemIDplus is a free, web search system that provides access to the structure and nomenclature authority files used for the identification of chemical substances cited in National Library of Medicine (NLM) databases, including the TOXNET system. | |
Record name | 2'-cyano-2'-deoxy-1-(beta-D-arabinofuranosyl)cytosine | |
Source | DrugBank | |
URL | https://www.drugbank.ca/drugs/DB11667 | |
Description | The DrugBank database is a unique bioinformatics and cheminformatics resource that combines detailed drug (i.e. chemical, pharmacological and pharmaceutical) data with comprehensive drug target (i.e. sequence, structure, and pathway) information. | |
Explanation | Creative Common's Attribution-NonCommercial 4.0 International License (http://creativecommons.org/licenses/by-nc/4.0/legalcode) | |
Record name | CNDAC | |
Source | EPA DSSTox | |
URL | https://comptox.epa.gov/dashboard/DTXSID30159474 | |
Description | DSSTox provides a high quality public chemistry resource for supporting improved predictive toxicology. | |
Record name | RADGOCITABINE | |
Source | FDA Global Substance Registration System (GSRS) | |
URL | https://gsrs.ncats.nih.gov/ginas/app/beta/substances/00M634HD2V | |
Description | The FDA Global Substance Registration System (GSRS) enables the efficient and accurate exchange of information on what substances are in regulated products. Instead of relying on names, which vary across regulatory domains, countries, and regions, the GSRS knowledge base makes it possible for substances to be defined by standardized, scientific descriptions. | |
Explanation | Unless otherwise noted, the contents of the FDA website (www.fda.gov), both text and graphics, are not copyrighted. They are in the public domain and may be republished, reprinted and otherwise used freely by anyone without the need to obtain permission from FDA. Credit to the U.S. Food and Drug Administration as the source is appreciated but not required. | |
Retrosynthesis Analysis
AI-Powered Synthesis Planning: Our tool employs the Template_relevance Pistachio, Template_relevance Bkms_metabolic, Template_relevance Pistachio_ringbreaker, Template_relevance Reaxys, Template_relevance Reaxys_biocatalysis model, leveraging a vast database of chemical reactions to predict feasible synthetic routes.
One-Step Synthesis Focus: Specifically designed for one-step synthesis, it provides concise and direct routes for your target compounds, streamlining the synthesis process.
Accurate Predictions: Utilizing the extensive PISTACHIO, BKMS_METABOLIC, PISTACHIO_RINGBREAKER, REAXYS, REAXYS_BIOCATALYSIS database, our tool offers high-accuracy predictions, reflecting the latest in chemical research and data.
Strategy Settings
Precursor scoring | Relevance Heuristic |
---|---|
Min. plausibility | 0.01 |
Model | Template_relevance |
Template Set | Pistachio/Bkms_metabolic/Pistachio_ringbreaker/Reaxys/Reaxys_biocatalysis |
Top-N result to add to graph | 6 |
Feasible Synthetic Routes
Q1: What is the primary mechanism of action of 2′-C-cyano-2′-deoxy-1-β-D-arabino-pentofuranosylcytosine (CNDAC)?
A: this compound, the active metabolite of sapacitabine, exerts its antitumor activity through a unique mechanism. After being phosphorylated to its triphosphate form (CNDACTP), it incorporates into DNA during replication. This incorporation triggers a β-elimination reaction, leading to the formation of single-strand breaks (SSBs) in the DNA. [, , , , ]
Q2: How do these single-strand breaks ultimately lead to cell death?
A: If left unrepaired, these SSBs are encountered by the replication machinery during the next cell cycle. This encounter leads to the conversion of SSBs into highly lethal double-strand breaks (DSBs). [, ]
Q3: How does this compound impact the cell cycle?
A: In addition to causing DNA damage, this compound also induces cell cycle arrest in the G2 phase. This arrest is attributed to the activation of the Chk1-Cdc25C-Cdk1/cyclin B checkpoint pathway, a critical signaling cascade that ensures proper DNA repair before cell division. [, ]
Q4: What is the role of homologous recombination (HR) in this compound's mechanism of action?
A: Homologous recombination (HR) is the primary DNA repair pathway responsible for repairing this compound-induced DSBs. [] Consequently, cells with deficiencies in HR components, such as ATM, Rad51, Xrcc3, and Brca2, exhibit heightened sensitivity to this compound. [, , , , , ]
Q5: What is the molecular formula and weight of this compound?
A5: The molecular formula of this compound is C10H12N4O4, and its molecular weight is 252.23 g/mol. [This information can be derived from the chemical name and is commonly found in chemical databases, but is not explicitly stated in the provided abstracts.]
Q6: What is known about the stability of this compound in different environments?
A: this compound undergoes epimerization to 2'-C-cyano-2'-deoxy-1-beta-D-ribo-pentofuranosylcytosine (CNDC) and degradation in alkaline media. This process is initiated by the abstraction of the acidic 2'-proton, leading to β-elimination reactions. [] These reactions also occur to a lesser extent at neutral pH and in cell culture media. []
Q7: How is this compound usually formulated for research and clinical use?
A: this compound itself and its prodrug, sapacitabine, can be formulated for oral administration. Additionally, this compound has been successfully incorporated into liposomal formulations, including those modified with targeting peptides, to improve its delivery and efficacy. [, , ]
Q8: What are the known mechanisms of resistance to this compound?
A8: Research suggests two main mechanisms for resistance:
- Deoxycytidine Kinase (dCK) Loss: Acquired resistance to this compound, as observed in this compound-adapted AML cell lines, is primarily driven by the loss of dCK, the enzyme responsible for the initial phosphorylation of this compound into its active form. []
- Increased SAMHD1 Levels: While this compound was initially thought to potentially inhibit SAMHD1, research has shown that SAMHD1 actually contributes to intrinsic resistance to this compound in leukemia cells by degrading this compound triphosphate (CNDACTP). []
Q9: Does resistance to this compound confer cross-resistance to other agents?
A: this compound-resistant cells primarily exhibit cross-resistance to other drugs that are substrates of dCK, such as cytarabine and decitabine. This specificity suggests that resistance mechanisms are often linked to the specific metabolic pathways of the drugs. [] Conversely, cells resistant to drugs not metabolized by dCK or SAMHD1 maintain their sensitivity to this compound. []
Q10: What in vitro models have been used to study this compound's activity?
A: Numerous studies have used a variety of human cancer cell lines, including leukemia (HL-60, THP-1, CCRF-CEM, ML-1, K562), ovarian (PEO1, UWB1.289), colon (HCT116), and gastric (TMK-1) carcinoma cell lines, to investigate this compound's efficacy. [, , , , , , , , , , , ] These models allow researchers to assess cell viability, cell cycle arrest, apoptosis induction, and DNA damage in response to this compound.
Q11: What in vivo models have been employed to evaluate this compound's efficacy?
A: this compound has shown potent antitumor activity in various human tumor xenograft models in mice, including models of leukemia (P388), sarcoma (Meth A), and lung metastasis. [, , ]
Q12: What are the key findings from clinical trials of sapacitabine?
A: Sapacitabine has shown promising clinical activity in patients with acute myeloid leukemia (AML) and myelodysplastic syndromes (MDS). [, , ] A Phase III trial for elderly AML patients and Phase II trials in AML, MDS, non-small cell lung cancer (NSCLC), and chronic lymphocytic leukemia (CLL)/small lymphocytic lymphoma (SLL) are underway. []
Q13: What drug delivery strategies have been explored to improve this compound's therapeutic index?
A: Liposomal formulations of this compound, particularly long-circulating liposomes modified with polyethylene glycol (PEG) and targeting peptides, have demonstrated enhanced antitumor efficacy and reduced toxicity compared to free this compound. [, , ] These modifications improve the pharmacokinetic properties of this compound, leading to increased tumor accumulation and reduced uptake by the reticuloendothelial system (RES).
Q14: Which targeting strategies have been explored for this compound liposomes?
A14: Two main targeting strategies have been explored:
- Angiogenic Vessel Targeting: Liposomes modified with peptides that bind to markers specifically expressed on angiogenic endothelial cells, such as APRPG and GPLPLR, have shown enhanced tumor accumulation and antitumor activity. [, ] This strategy exploits the dependence of tumors on angiogenesis for growth and metastasis.
- Passive Tumor Targeting: Long-circulating liposomes modified with PEG and incorporating 5'-O-dipalmitoylphosphatidyl this compound (DPP-CNDAC) have shown enhanced tumor accumulation due to the enhanced permeability and retention (EPR) effect. [, ] This passive targeting takes advantage of the leaky vasculature and impaired lymphatic drainage often observed in tumors.
Q15: Are there any potential biomarkers for predicting response to this compound or sapacitabine?
A:
HR Deficiency: Given the central role of HR in repairing this compound-induced DNA damage, defects in HR pathway components, including BRCA1/2, Rad51, XRCC3, and ATM, are likely to sensitize tumor cells to this compound and may serve as potential biomarkers for treatment response. [, , , , , ]* BER and TDP1:* While HR deficiency is a major predictor of response, research also suggests a role for base excision repair (BER) and Tyrosyl-DNA phosphodiesterase 1 (TDP1) in repairing this compound-induced DNA lesions. Defects in these pathways could also potentially sensitize cells to this compound. [, ]
Descargo de responsabilidad e información sobre productos de investigación in vitro
Tenga en cuenta que todos los artículos e información de productos presentados en BenchChem están destinados únicamente con fines informativos. Los productos disponibles para la compra en BenchChem están diseñados específicamente para estudios in vitro, que se realizan fuera de organismos vivos. Los estudios in vitro, derivados del término latino "in vidrio", involucran experimentos realizados en entornos de laboratorio controlados utilizando células o tejidos. Es importante tener en cuenta que estos productos no se clasifican como medicamentos y no han recibido la aprobación de la FDA para la prevención, tratamiento o cura de ninguna condición médica, dolencia o enfermedad. Debemos enfatizar que cualquier forma de introducción corporal de estos productos en humanos o animales está estrictamente prohibida por ley. Es esencial adherirse a estas pautas para garantizar el cumplimiento de los estándares legales y éticos en la investigación y experimentación.