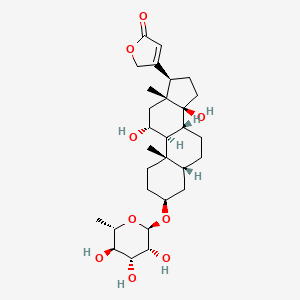
Rhodexin A
Descripción general
Descripción
Rhodexin A is a cardiac glycoside first isolated from the leaves and roots of Rhodea Japonica in 1951 . It has shown potent activity against leukemia K562 cells with an IC50 value of 19 nanomolar . This compound is characterized by its unique geometry at the AB and CD ring junctures, displaying a cis rather than a trans fusion, a tertiary hydroxyl group at carbon 14, and a beta-butenolide moiety at carbon 17 .
Métodos De Preparación
Synthetic Routes and Reaction Conditions: The enantiospecific total synthesis of Rhodexin A involves a very hindered inverse electron demand Diels-Alder reaction . The key step in the synthesis is the reaction of the very hindered dienone and the silyl enol ether catalyzed by the strong Lewis acid, dimethylaluminum triflimide . The synthesis begins with the preparation of the tetracyclic core of this compound, which involves the stepwise Diels-Alder reaction followed by a Mukaiyama Michael reaction and a Mukaiyama aldol process .
Industrial Production Methods: Industrial production methods for this compound are not well-documented in the literature. the synthetic routes developed in academic research can potentially be scaled up for industrial production, provided that the reaction conditions and catalysts are optimized for large-scale synthesis.
Análisis De Reacciones Químicas
Types of Reactions: Rhodexin A undergoes various types of chemical reactions, including:
Oxidation: The oxidation of this compound can lead to the formation of different oxidation products depending on the reagents and conditions used.
Reduction: Reduction reactions can modify the functional groups present in this compound, such as the beta-butenolide moiety.
Substitution: Substitution reactions can occur at various positions on the this compound molecule, leading to the formation of different derivatives.
Common Reagents and Conditions:
Oxidation: Common oxidizing agents include potassium permanganate and chromium trioxide.
Reduction: Common reducing agents include lithium aluminum hydride and sodium borohydride.
Substitution: Common reagents for substitution reactions include halogens and nucleophiles.
Major Products: The major products formed from these reactions depend on the specific reagents and conditions used. For example, oxidation reactions can lead to the formation of oxidized derivatives, while reduction reactions can produce reduced forms of this compound.
Aplicaciones Científicas De Investigación
Rhodexin A has several scientific research applications, including:
Chemistry: this compound is used as a model compound for studying the synthesis and reactivity of cardiac glycosides.
Industry: this compound can be used in the development of new drugs and therapeutic agents due to its unique chemical structure and biological activity.
Mecanismo De Acción
The mechanism of action of Rhodexin A involves its interaction with molecular targets and pathways in the body. This compound inhibits the synthesis of hypoxia-inducible factor 1 alpha, which is a key regulator of cellular response to low oxygen levels . This inhibition leads to the antiproliferative effects observed in leukemia cells. Additionally, this compound exerts its cardiotonic effects by interacting with the sodium-potassium ATPase pump, which regulates the concentration of sodium and potassium ions in cardiac cells .
Comparación Con Compuestos Similares
Sarmentogenin: Rhodexin A is the L-rhamnoside of sarmentogenin.
Rhodexoside: A diglycoside of sarmentogenin, which includes rhamnose and glucose.
Uniqueness: this compound is unique due to its cis fusion at the AB and CD ring junctures, the presence of a tertiary hydroxyl group at carbon 14, and a beta-butenolide moiety at carbon 17 . These structural features contribute to its distinct biological activity and make it an attractive target for synthetic and medicinal chemistry research.
Actividad Biológica
Rhodexin A is a cardiac glycoside first isolated from the leaves and roots of Rohdea japonica in 1951. It is characterized by its unique structural features, including an unusual geometry at the AB and CD ring junctures, which exhibit a cis fusion rather than the typical trans configuration. This compound has garnered significant interest due to its potent biological activities, particularly its antiproliferative effects against human leukemia cells.
Chemical Structure and Synthesis
This compound has a complex tetracyclic structure with specific functional groups that contribute to its biological activity. The total synthesis of this compound has been achieved through innovative synthetic methodologies, including inverse electron-demand Diels-Alder reactions, which facilitate the formation of the required stereocenters in a single step .
Key Structural Features
- Cis Fusion : The AB and CD rings display a cis configuration.
- Tertiary Hydroxyl Group : Present at C14.
- β-Butenolide Moiety : Located at C17.
Synthetic Pathway Overview
The synthesis involves several steps:
- Formation of Cycloadducts : Initial reactions yield cycloadducts with the desired stereochemistry.
- Elaboration of Tetracyclic Core : Subsequent transformations lead to the fully functionalized tetracyclic core of this compound.
- Final Modifications : Additional steps refine the structure to achieve this compound .
Biological Activity
This compound exhibits significant biological activity, particularly as an antiproliferative agent against various cancer cell lines. Notably, it has shown potent inhibitory effects on human leukemia K562 cells with an IC50 value of 19 nM, indicating high efficacy .
The biological activity of this compound is primarily attributed to its ability to inhibit the synthesis of hypoxia-inducible factor 1 (HIF-1α), a crucial regulator in cancer cell proliferation and survival. This inhibition leads to:
- Cell Cycle Arrest : this compound induces cell cycle arrest in cancer cells.
- Apoptosis : It triggers programmed cell death, further contributing to its antiproliferative effects .
Summary of Biological Studies
Study | Cell Line | IC50 (nM) | Mechanism |
---|---|---|---|
Study 1 | K562 (human leukemia) | 19 | Inhibition of HIF-1α |
Study 2 | Various cancer lines | Varies | Induces apoptosis |
Case Studies and Research Findings
Several studies have highlighted the potential of this compound in cancer therapy:
- Flow Cytometric Analysis : This study demonstrated that this compound effectively inhibits the growth of K562 cells by inducing both cell cycle arrest and apoptosis. Flow cytometry was used to quantify these effects, confirming its role as a potent anticancer agent .
- Comparative Studies : Research comparing this compound with other cardiac glycosides showed that it possesses superior antiproliferative properties, making it a candidate for further drug development in oncology .
- Mechanistic Insights : Further investigations into its mechanism revealed that this compound affects multiple signaling pathways involved in cell survival and proliferation, suggesting potential combinatory therapies with existing cancer treatments .
Q & A
Q. Basic: How is Rhodexin A structurally characterized, and what analytical techniques are essential for its identification?
Answer:
this compound, a cardiac glycoside, is identified via high-resolution mass spectrometry (HR-MS) for molecular weight determination (e.g., C₃₅H₅₄O₁₄ for rhodexin C analogs) and nuclear magnetic resonance (NMR) spectroscopy for elucidating stereochemistry and functional groups. Polarimetry ([α] values) and melting point analysis (e.g., decomposition at 75°C for rhodexin C) are critical for purity assessment . Researchers must cross-reference spectral data with literature and validate findings using authenticated reference standards to avoid misidentification .
Q. Basic: What methodologies are recommended for isolating this compound from plant sources like Rohdea japonica?
Answer:
Isolation involves solvent extraction (e.g., ethanol/water mixtures), followed by liquid-liquid partitioning and chromatographic techniques (e.g., column chromatography with silica gel or Sephadex LH-20). Advanced purification employs preparative HPLC with C18 columns. Document solvent ratios, temperature, and pressure parameters to ensure reproducibility. For novel protocols, provide step-by-step validation, including yield calculations and purity thresholds (≥95% by HPLC) .
Q. Advanced: How can researchers resolve contradictory pharmacological data for this compound across in vitro and in vivo models?
Answer:
Contradictions often arise from differences in bioavailability, metabolic pathways, or model-specific variables (e.g., cell line vs. animal species). Address discrepancies by:
- Conducting dose-response studies across multiple models.
- Validating target engagement via biochemical assays (e.g., Na⁺/K⁺-ATPase inhibition for cardiac glycosides).
- Performing pharmacokinetic (PK) analyses to correlate plasma concentrations with efficacy/toxicity .
Report negative results transparently and discuss confounding factors (e.g., matrix effects in plant extracts) .
Q. Advanced: What experimental designs are optimal for studying this compound’s pharmacokinetics (ADME) in preclinical models?
Answer:
Use radiolabeled this compound (³H/¹⁴C) for precise absorption, distribution, metabolism, and excretion (ADME) tracking. Employ LC-MS/MS for quantifying parent compounds and metabolites in plasma/tissue. Design studies with:
- Temporal sampling: Collect data at multiple time points to model half-life and bioavailability.
- Cross-species validation: Compare rodent and non-rodent models to assess translational relevance.
- Tissue-specific analysis: Prioritize organs implicated in toxicity (e.g., heart, liver) .
Q. Basic: How should researchers assess the purity and stability of this compound in experimental preparations?
Answer:
Purity is quantified via HPLC-UV (λ = 220–280 nm for glycosides) with ≥95% peak area threshold. Stability studies require:
- Forced degradation: Expose this compound to heat, light, and pH extremes to identify degradation products.
- Long-term storage: Monitor compound integrity at -80°C over 6–12 months using validated assays.
Document all conditions in supplementary materials to ensure reproducibility .
Q. Advanced: What strategies mitigate batch-to-batch variability in this compound derived from natural sources?
Answer:
Variability arises from seasonal, geographical, or extraction inconsistencies. Mitigation strategies include:
- Standardized cultivation: Control growth conditions (soil pH, light exposure) for source plants.
- Quality-by-design (QbD): Optimize extraction parameters (e.g., solvent polarity, temperature) using statistical modeling.
- Synthetic analogs: Develop semi-synthetic derivatives to bypass natural product variability .
Q. Basic: What safety protocols are essential when handling this compound in laboratory settings?
Answer:
Follow GHS guidelines:
- Personal protective equipment (PPE): Gloves, lab coats, and goggles to prevent skin/eye contact.
- Ventilation: Use fume hoods to avoid inhalation of aerosols.
- Emergency procedures: Immediate rinsing for spills (15+ minutes for eye exposure) and medical consultation for ingestion .
Q. Advanced: How can computational modeling enhance the study of this compound’s mechanism of action?
Answer:
Molecular docking (e.g., AutoDock Vina) predicts binding affinities to targets like Na⁺/K⁺-ATPase. Molecular dynamics simulations (e.g., GROMACS) assess conformational stability. Validate predictions with mutational studies (e.g., alanine scanning) and biophysical assays (e.g., surface plasmon resonance) .
Propiedades
IUPAC Name |
3-[(3S,5R,8R,9S,10S,11R,13R,14S,17R)-11,14-dihydroxy-10,13-dimethyl-3-[(2R,3R,4R,5R,6S)-3,4,5-trihydroxy-6-methyloxan-2-yl]oxy-1,2,3,4,5,6,7,8,9,11,12,15,16,17-tetradecahydrocyclopenta[a]phenanthren-17-yl]-2H-furan-5-one | |
---|---|---|
Source | PubChem | |
URL | https://pubchem.ncbi.nlm.nih.gov | |
Description | Data deposited in or computed by PubChem | |
InChI |
InChI=1S/C29H44O9/c1-14-23(32)24(33)25(34)26(37-14)38-17-6-8-27(2)16(11-17)4-5-19-22(27)20(30)12-28(3)18(7-9-29(19,28)35)15-10-21(31)36-13-15/h10,14,16-20,22-26,30,32-35H,4-9,11-13H2,1-3H3/t14-,16+,17-,18+,19+,20+,22+,23-,24+,25+,26-,27-,28+,29-/m0/s1 | |
Source | PubChem | |
URL | https://pubchem.ncbi.nlm.nih.gov | |
Description | Data deposited in or computed by PubChem | |
InChI Key |
HFMLTKBZNAPPNY-CEKKCSHUSA-N | |
Source | PubChem | |
URL | https://pubchem.ncbi.nlm.nih.gov | |
Description | Data deposited in or computed by PubChem | |
Canonical SMILES |
CC1C(C(C(C(O1)OC2CCC3(C(C2)CCC4C3C(CC5(C4(CCC5C6=CC(=O)OC6)O)C)O)C)O)O)O | |
Source | PubChem | |
URL | https://pubchem.ncbi.nlm.nih.gov | |
Description | Data deposited in or computed by PubChem | |
Isomeric SMILES |
C[C@H]1[C@@H]([C@H]([C@H]([C@@H](O1)O[C@H]2CC[C@]3([C@@H](C2)CC[C@@H]4[C@@H]3[C@@H](C[C@]5([C@@]4(CC[C@@H]5C6=CC(=O)OC6)O)C)O)C)O)O)O | |
Source | PubChem | |
URL | https://pubchem.ncbi.nlm.nih.gov | |
Description | Data deposited in or computed by PubChem | |
Molecular Formula |
C29H44O9 | |
Source | PubChem | |
URL | https://pubchem.ncbi.nlm.nih.gov | |
Description | Data deposited in or computed by PubChem | |
DSSTOX Substance ID |
DTXSID301318334 | |
Record name | Rhodexin A | |
Source | EPA DSSTox | |
URL | https://comptox.epa.gov/dashboard/DTXSID301318334 | |
Description | DSSTox provides a high quality public chemistry resource for supporting improved predictive toxicology. | |
Molecular Weight |
536.7 g/mol | |
Source | PubChem | |
URL | https://pubchem.ncbi.nlm.nih.gov | |
Description | Data deposited in or computed by PubChem | |
CAS No. |
545-49-3 | |
Record name | Rhodexin A | |
Source | CAS Common Chemistry | |
URL | https://commonchemistry.cas.org/detail?cas_rn=545-49-3 | |
Description | CAS Common Chemistry is an open community resource for accessing chemical information. Nearly 500,000 chemical substances from CAS REGISTRY cover areas of community interest, including common and frequently regulated chemicals, and those relevant to high school and undergraduate chemistry classes. This chemical information, curated by our expert scientists, is provided in alignment with our mission as a division of the American Chemical Society. | |
Explanation | The data from CAS Common Chemistry is provided under a CC-BY-NC 4.0 license, unless otherwise stated. | |
Record name | Rhodexin A | |
Source | ChemIDplus | |
URL | https://pubchem.ncbi.nlm.nih.gov/substance/?source=chemidplus&sourceid=0000545493 | |
Description | ChemIDplus is a free, web search system that provides access to the structure and nomenclature authority files used for the identification of chemical substances cited in National Library of Medicine (NLM) databases, including the TOXNET system. | |
Record name | Rhodexin A | |
Source | EPA DSSTox | |
URL | https://comptox.epa.gov/dashboard/DTXSID301318334 | |
Description | DSSTox provides a high quality public chemistry resource for supporting improved predictive toxicology. | |
Retrosynthesis Analysis
AI-Powered Synthesis Planning: Our tool employs the Template_relevance Pistachio, Template_relevance Bkms_metabolic, Template_relevance Pistachio_ringbreaker, Template_relevance Reaxys, Template_relevance Reaxys_biocatalysis model, leveraging a vast database of chemical reactions to predict feasible synthetic routes.
One-Step Synthesis Focus: Specifically designed for one-step synthesis, it provides concise and direct routes for your target compounds, streamlining the synthesis process.
Accurate Predictions: Utilizing the extensive PISTACHIO, BKMS_METABOLIC, PISTACHIO_RINGBREAKER, REAXYS, REAXYS_BIOCATALYSIS database, our tool offers high-accuracy predictions, reflecting the latest in chemical research and data.
Strategy Settings
Precursor scoring | Relevance Heuristic |
---|---|
Min. plausibility | 0.01 |
Model | Template_relevance |
Template Set | Pistachio/Bkms_metabolic/Pistachio_ringbreaker/Reaxys/Reaxys_biocatalysis |
Top-N result to add to graph | 6 |
Feasible Synthetic Routes
Descargo de responsabilidad e información sobre productos de investigación in vitro
Tenga en cuenta que todos los artículos e información de productos presentados en BenchChem están destinados únicamente con fines informativos. Los productos disponibles para la compra en BenchChem están diseñados específicamente para estudios in vitro, que se realizan fuera de organismos vivos. Los estudios in vitro, derivados del término latino "in vidrio", involucran experimentos realizados en entornos de laboratorio controlados utilizando células o tejidos. Es importante tener en cuenta que estos productos no se clasifican como medicamentos y no han recibido la aprobación de la FDA para la prevención, tratamiento o cura de ninguna condición médica, dolencia o enfermedad. Debemos enfatizar que cualquier forma de introducción corporal de estos productos en humanos o animales está estrictamente prohibida por ley. Es esencial adherirse a estas pautas para garantizar el cumplimiento de los estándares legales y éticos en la investigación y experimentación.