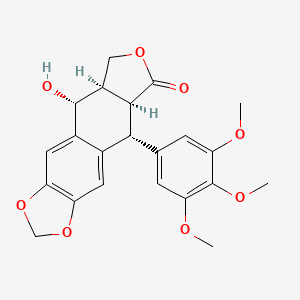
Picropodofilina
Descripción general
Descripción
Picropodophyllin is a naturally occurring aryltetralin lignan found in the roots of the Podophyllum hexandrum plant. It is an epimer of podophyllotoxin and exhibits significant biological activities, including antitumor, antifungal, and insecticidal properties . This compound has garnered attention for its potential therapeutic applications, particularly in cancer treatment.
Mecanismo De Acción
Target of Action
Picropodophyllin (PPP), also known as picropodophyllotoxin, primarily targets the Insulin-like Growth Factor-1 Receptor (IGF-1R) . IGF-1R is a receptor tyrosine kinase that plays a crucial role in cell growth, differentiation, and survival . Overexpression of IGF-1R has been associated with various types of cancers, making it a potential target for anticancer therapy .
Mode of Action
PPP acts as a potent inhibitor of IGF-1R . It interferes with the receptor’s activity, leading to a blockage of the downstream signaling pathways . This blockage results in the inhibition of cancer cell proliferation, migration, adhesion, and cell cycle progression .
Biochemical Pathways
PPP’s inhibition of IGF-1R affects several downstream signaling pathways. One of the key pathways influenced is the Mitogen-Activated Protein Kinase (MAPK) pathway . PPP’s action attenuates MAPK phosphorylation, leading to cell cycle arrest in the G2/M phase . This disruption of the cell cycle is a significant factor in PPP’s antitumor effects.
Result of Action
PPP’s action leads to significant molecular and cellular effects. It strongly inhibits cancer cell proliferation and induces apoptosis in certain cell lines . Additionally, PPP causes cell cycle arrest, specifically in the G2/M phase . These effects contribute to PPP’s potential as an anticancer agent.
Action Environment
It’s known that ppp’s efficacy can vary depending on the specific type of cancer cells and their igf-1r expression levels
Aplicaciones Científicas De Investigación
Picropodophyllin has a wide range of scientific research applications:
Chemistry: It is used as a precursor for synthesizing other biologically active compounds.
Biology: It serves as a tool for studying cell cycle regulation and apoptosis.
Industry: It is used in the development of insecticidal and antifungal agents.
Análisis Bioquímico
Biochemical Properties
Picropodophyllotoxin plays a crucial role in biochemical reactions, particularly in the context of its antitumor activity. It interacts with several enzymes, proteins, and other biomolecules. Notably, picropodophyllotoxin targets tubulin and topoisomerase II, leading to cell cycle arrest at the G2/M phase and inducing DNA breaks . These interactions are essential for its ability to inhibit cancer cell proliferation.
Cellular Effects
Picropodophyllotoxin exerts significant effects on various types of cells and cellular processes. It induces apoptosis in human esophageal squamous cell carcinoma cells through reactive oxygen species (ROS)-mediated activation of the c-Jun N-terminal kinase (JNK) and p38 mitogen-activated protein kinase (MAPK) pathways . This compound also influences cell signaling pathways, gene expression, and cellular metabolism, leading to the inhibition of cell viability and the induction of cell cycle arrest .
Molecular Mechanism
The molecular mechanism of picropodophyllotoxin involves its binding interactions with biomolecules, enzyme inhibition, and changes in gene expression. Picropodophyllotoxin binds to the colchicine binding site of tubulin, inhibiting microtubule assembly and leading to cell cycle arrest . Additionally, it induces apoptosis by increasing ROS levels and activating the JNK/p38 signaling pathways . These molecular interactions are critical for its antitumor activity.
Temporal Effects in Laboratory Settings
In laboratory settings, the effects of picropodophyllotoxin change over time. Studies have shown that picropodophyllotoxin inhibits the viability of esophageal squamous cell carcinoma cells in a time-dependent manner . The compound’s stability and degradation over time can influence its long-term effects on cellular function. In vitro and in vivo studies have demonstrated that picropodophyllotoxin induces apoptosis and cell cycle arrest over extended periods .
Dosage Effects in Animal Models
The effects of picropodophyllotoxin vary with different dosages in animal models. Higher doses of picropodophyllotoxin have been associated with increased apoptosis and cell cycle arrest in cancer cells . At high doses, picropodophyllotoxin may also exhibit toxic or adverse effects, highlighting the importance of determining optimal dosage levels for therapeutic use .
Metabolic Pathways
Picropodophyllotoxin is involved in several metabolic pathways, including those related to its antitumor activity. It interacts with enzymes such as topoisomerase II and tubulin, leading to the inhibition of DNA replication and cell division . These interactions are crucial for its ability to induce apoptosis and inhibit cancer cell proliferation.
Transport and Distribution
Within cells and tissues, picropodophyllotoxin is transported and distributed through interactions with various transporters and binding proteins. These interactions influence its localization and accumulation within specific cellular compartments . The compound’s distribution is essential for its effectiveness in targeting cancer cells.
Subcellular Localization
Picropodophyllotoxin’s subcellular localization plays a significant role in its activity and function. It is primarily localized in the cytoplasm, where it interacts with tubulin and other proteins involved in cell division . This localization is critical for its ability to inhibit microtubule assembly and induce cell cycle arrest.
Métodos De Preparación
Synthetic Routes and Reaction Conditions
Picropodophyllin can be synthesized through various chemical routes. One common method involves the biotransformation of podophyllotoxin using specific bacterial strains such as Pseudomonas aeruginosa . This process involves the isomerization and hydrolysis of podophyllotoxin to produce picropodophyllotoxin and podophyllic acid.
Industrial Production Methods
Industrial production of picropodophyllotoxin typically involves the extraction and purification from the roots of Podophyllum hexandrum. The extraction process includes solvent extraction, followed by chromatographic techniques to isolate and purify the compound .
Análisis De Reacciones Químicas
Types of Reactions
Picropodophyllin undergoes various chemical reactions, including:
Oxidation: It can be oxidized to form different derivatives.
Reduction: Reduction reactions can modify its functional groups.
Substitution: Substitution reactions can introduce new functional groups into the molecule.
Common Reagents and Conditions
Common reagents used in these reactions include oxidizing agents like potassium permanganate and reducing agents like sodium borohydride. Reaction conditions vary depending on the desired product but often involve controlled temperatures and pH levels .
Major Products Formed
The major products formed from these reactions include various derivatives of picropodophyllotoxin, which can exhibit different biological activities. For example, oxidation can lead to the formation of podophyllic acid .
Comparación Con Compuestos Similares
Similar Compounds
Podophyllotoxin: An epimer of picropodophyllotoxin with similar biological activities.
Etoposide: A derivative of podophyllotoxin used as a chemotherapeutic agent.
Teniposide: Another podophyllotoxin derivative with anticancer properties.
Uniqueness
Picropodophyllin is unique due to its specific mechanism of action and its ability to selectively induce apoptosis in cancer cells through the JNK/p38 MAPK pathways . This makes it a promising candidate for developing targeted anticancer therapies.
Propiedades
IUPAC Name |
5-hydroxy-9-(3,4,5-trimethoxyphenyl)-5a,6,8a,9-tetrahydro-5H-[2]benzofuro[5,6-f][1,3]benzodioxol-8-one | |
---|---|---|
Source | PubChem | |
URL | https://pubchem.ncbi.nlm.nih.gov | |
Description | Data deposited in or computed by PubChem | |
InChI |
InChI=1S/C22H22O8/c1-25-16-4-10(5-17(26-2)21(16)27-3)18-11-6-14-15(30-9-29-14)7-12(11)20(23)13-8-28-22(24)19(13)18/h4-7,13,18-20,23H,8-9H2,1-3H3 | |
Source | PubChem | |
URL | https://pubchem.ncbi.nlm.nih.gov | |
Description | Data deposited in or computed by PubChem | |
InChI Key |
YJGVMLPVUAXIQN-UHFFFAOYSA-N | |
Source | PubChem | |
URL | https://pubchem.ncbi.nlm.nih.gov | |
Description | Data deposited in or computed by PubChem | |
Canonical SMILES |
COC1=CC(=CC(=C1OC)OC)C2C3C(COC3=O)C(C4=CC5=C(C=C24)OCO5)O | |
Source | PubChem | |
URL | https://pubchem.ncbi.nlm.nih.gov | |
Description | Data deposited in or computed by PubChem | |
Molecular Formula |
C22H22O8 | |
Source | PubChem | |
URL | https://pubchem.ncbi.nlm.nih.gov | |
Description | Data deposited in or computed by PubChem | |
DSSTOX Substance ID |
DTXSID301347791 | |
Record name | 5,8,8a,9-Tetrahydro-9-hydroxy-5-(3,4,5-trimethoxyphenyl)furo(3',4':6,7)naphtho(2,3-d)-1,3-dioxol-6(5aH)-one | |
Source | EPA DSSTox | |
URL | https://comptox.epa.gov/dashboard/DTXSID301347791 | |
Description | DSSTox provides a high quality public chemistry resource for supporting improved predictive toxicology. | |
Molecular Weight |
414.4 g/mol | |
Source | PubChem | |
URL | https://pubchem.ncbi.nlm.nih.gov | |
Description | Data deposited in or computed by PubChem | |
CAS No. |
4354-76-1 | |
Record name | Furo(3',4':6,7)naphtho(2,3-d)-1,3-dioxol-6(5aH)-one, 5,8,8a,9-tetrahydro-9-hydroxy-5-(3,4,5-trimethoxyphenyl)- | |
Source | ChemIDplus | |
URL | https://pubchem.ncbi.nlm.nih.gov/substance/?source=chemidplus&sourceid=0004354761 | |
Description | ChemIDplus is a free, web search system that provides access to the structure and nomenclature authority files used for the identification of chemical substances cited in National Library of Medicine (NLM) databases, including the TOXNET system. | |
Record name | 5,8,8a,9-Tetrahydro-9-hydroxy-5-(3,4,5-trimethoxyphenyl)furo(3',4':6,7)naphtho(2,3-d)-1,3-dioxol-6(5aH)-one | |
Source | EPA DSSTox | |
URL | https://comptox.epa.gov/dashboard/DTXSID301347791 | |
Description | DSSTox provides a high quality public chemistry resource for supporting improved predictive toxicology. | |
Retrosynthesis Analysis
AI-Powered Synthesis Planning: Our tool employs the Template_relevance Pistachio, Template_relevance Bkms_metabolic, Template_relevance Pistachio_ringbreaker, Template_relevance Reaxys, Template_relevance Reaxys_biocatalysis model, leveraging a vast database of chemical reactions to predict feasible synthetic routes.
One-Step Synthesis Focus: Specifically designed for one-step synthesis, it provides concise and direct routes for your target compounds, streamlining the synthesis process.
Accurate Predictions: Utilizing the extensive PISTACHIO, BKMS_METABOLIC, PISTACHIO_RINGBREAKER, REAXYS, REAXYS_BIOCATALYSIS database, our tool offers high-accuracy predictions, reflecting the latest in chemical research and data.
Strategy Settings
Precursor scoring | Relevance Heuristic |
---|---|
Min. plausibility | 0.01 |
Model | Template_relevance |
Template Set | Pistachio/Bkms_metabolic/Pistachio_ringbreaker/Reaxys/Reaxys_biocatalysis |
Top-N result to add to graph | 6 |
Feasible Synthetic Routes
Q1: What is the primary mechanism of action of Picropodophyllotoxin?
A1: Picropodophyllotoxin (PPT) primarily exerts its anticancer activity by inhibiting microtubule assembly. It binds to tubulin, the building block of microtubules, disrupting their formation and dynamics. This interference with microtubule function disrupts essential cellular processes like mitosis, ultimately leading to cell cycle arrest and apoptosis [, , ].
Q2: How does PPT's mechanism differ from other podophyllotoxin derivatives like etoposide?
A2: While PPT targets microtubules, some derivatives like etoposide lack this activity []. Etoposide exerts its anticancer effects primarily by inhibiting topoisomerase II, an enzyme essential for DNA replication and repair. This distinction highlights the diverse mechanisms of action within the podophyllotoxin family.
Q3: Does PPT impact other signaling pathways besides those related to microtubules?
A3: Research suggests that PPT can influence multiple signaling pathways. For instance, studies show it can induce apoptosis in esophageal squamous cell carcinoma cells through reactive oxygen species (ROS) generation and activation of the JNK/p38 MAPK pathways []. In human colorectal cancer cells, PPT was found to induce G1 cell cycle arrest and apoptosis, also via ROS generation and activation of the p38 MAPK signaling pathway [].
Q4: What are the downstream effects of PPT's interaction with its targets?
A5: The downstream effects of PPT depend on its target. When PPT binds to tubulin, it disrupts microtubule dynamics, leading to mitotic arrest, ultimately triggering apoptotic pathways [, , ]. When interacting with receptors like EGFR and MET, PPT can inhibit their downstream signaling, impacting cell proliferation and survival []. Further research is needed to fully elucidate the complete range of downstream effects and their implications in different cellular contexts.
Q5: What is the molecular formula and weight of PPT?
A5: The molecular formula of PPT is C22H22O8, and its molecular weight is 414.40 g/mol.
Q6: Are there specific spectroscopic data characteristics of PPT?
A7: Yes, PPT can be characterized using techniques like nuclear magnetic resonance (NMR) spectroscopy and mass spectrometry (MS). Studies have employed these techniques to analyze PPT and its derivatives, providing valuable structural information [, , ]. For example, EI mass spectrometry can distinguish between the 2-epimers of podophyllotoxin and its esters, including PPT, by analyzing the relative abundance of specific fragment ions [, ].
Q7: How do structural modifications of PPT affect its activity?
A8: SAR studies have illuminated the relationship between PPT's structure and its activity. For example, the presence of a free hydroxyl group at the 4'-position appears essential for DNA breakage activity []. Epimerization at the 4-position of the podophyllotoxin rings enhances activity, while glucosylation at the 4-position diminishes it [, ]. These findings highlight how subtle structural changes can significantly impact PPT's biological activity.
Q8: Are there structural modifications that improve PPT's potency or selectivity?
A9: Research suggests that certain modifications can influence PPT's potency and selectivity. For example, in a study investigating insecticidal activity, podophyllotoxin exhibited higher potency than PPT and epipodophyllotoxin. Additionally, the 4'-hydroxyl series showed lower insecticidal activity compared to the 4'-oxymethyl series []. Further investigations into specific structural modifications could lead to the development of more potent and selective PPT analogs for therapeutic applications.
Q9: What cell lines have been used to study PPT's in vitro efficacy?
A9: PPT's in vitro efficacy has been investigated in various cancer cell lines, including:
- Human esophageal squamous cell carcinoma cells (KYSE 30 and KYSE 450) []
- Human colorectal cancer cells (HCT116) []
- Gefitinib-resistant non-small lung cancer cells (HCC827GR) []
- Human promyeloid leukemic HL-60 cells []
- HeLa, CNE, SH-SY5Y, and K562 cell lines []
Q10: Have any animal models been used to study PPT's in vivo activity?
A11: Yes, PPT's anti-angiogenic activity was studied using a chick chorioallantoic membrane (CAM) model []. Additionally, a study investigating the compound's effect on colon DNA damage utilized athymic mice injected with GH-secreting xenografts []. These models provide valuable insights into PPT's potential therapeutic effects in vivo.
Q11: What analytical methods are used to characterize and quantify PPT?
A11: Common analytical techniques used to characterize and quantify PPT include:
- High-Performance Liquid Chromatography (HPLC): This method is widely used to separate, identify, and quantify PPT and its derivatives in various matrices [, , ].
- Mass Spectrometry (MS): MS techniques like EI-MS are valuable for structural analysis and identifying specific PPT derivatives based on their fragmentation patterns [, ].
- Nuclear Magnetic Resonance (NMR) Spectroscopy: NMR provides detailed structural information about PPT, aiding in its identification and characterization [, ].
Q12: Has any research focused on developing specific analytical methods for PPT?
A15: Yes, a study focused on developing a reverse-phase HPLC (RP-HPLC) method for the simultaneous determination of PPT, deoxypodophyllotoxin, and podophyllotoxin in Sabina vulgaris []. This method demonstrated good accuracy, precision, and sensitivity for quantifying these compounds in plant extracts.
Q13: Is there information about PPT's stability under various conditions?
A16: While specific data on PPT's stability might be limited in the provided research, a study explored the influence of acids and bases on the γ-lactone and C-2 configuration of podophyllotoxin []. Understanding the stability of PPT under different pH conditions is crucial for developing suitable formulations and storage conditions.
Q14: Are there studies exploring the biotransformation of PPT?
A17: Yes, researchers have explored the biotransformation of PPT using microbial and plant cell cultures [, , , ]. For instance, a study found that cell suspension cultures of Rheum palmatum could convert podophyllotoxin to PPT, while root cultures could generate epipodophyllotoxin and apopodophyllotoxin []. These biotransformation approaches may offer sustainable routes for producing PPT and other valuable derivatives.
Q15: What are the potential implications of PPT's interaction with IGF-1 signaling?
A18: While research on PPT's direct interaction with IGF-1 signaling is limited, studies highlight the importance of this pathway. For example, disruption of IGF-1 signaling in astrocytes was found to affect glutamate handling, potentially contributing to neurodegeneration []. Understanding the interplay between PPT and IGF-1 signaling could be crucial for elucidating its effects on cell growth, survival, and potential implications in various diseases.
Descargo de responsabilidad e información sobre productos de investigación in vitro
Tenga en cuenta que todos los artículos e información de productos presentados en BenchChem están destinados únicamente con fines informativos. Los productos disponibles para la compra en BenchChem están diseñados específicamente para estudios in vitro, que se realizan fuera de organismos vivos. Los estudios in vitro, derivados del término latino "in vidrio", involucran experimentos realizados en entornos de laboratorio controlados utilizando células o tejidos. Es importante tener en cuenta que estos productos no se clasifican como medicamentos y no han recibido la aprobación de la FDA para la prevención, tratamiento o cura de ninguna condición médica, dolencia o enfermedad. Debemos enfatizar que cualquier forma de introducción corporal de estos productos en humanos o animales está estrictamente prohibida por ley. Es esencial adherirse a estas pautas para garantizar el cumplimiento de los estándares legales y éticos en la investigación y experimentación.