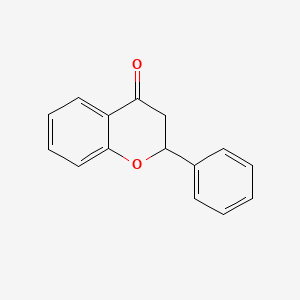
Flavanona
Descripción general
Descripción
La flavanona es un tipo de flavonoide, que es una clase de compuestos polifenólicos que se encuentran en diversas plantas. Estos compuestos son conocidos por su estructura aromática, cetónica e incolora derivada de la flavona. Las flavanonas a menudo se presentan en las plantas como glucósidos y se distribuyen ampliamente en los cítricos. Desempeñan un papel importante en el metabolismo vegetal y tienen diversas actividades biológicas, incluidas las propiedades antioxidantes, antiinflamatorias y anticancerígenas .
Aplicaciones Científicas De Investigación
Las flavanonas tienen una amplia gama de aplicaciones en la investigación científica:
Química: Utilizadas como intermedias en la síntesis de otros flavonoides y compuestos relacionados.
Biología: Estudiadas por su papel en el metabolismo vegetal y los mecanismos de defensa.
Medicina: Investigadas por sus potenciales efectos terapéuticos, incluidas las actividades antioxidantes, antiinflamatorias y anticancerígenas
Industria: Utilizadas como antioxidantes naturales en productos alimenticios y cosméticos.
Mecanismo De Acción
Las flavanonas ejercen sus efectos a través de diversos mecanismos. Pueden interactuar con múltiples dianas moleculares, incluyendo enzimas y receptores. Por ejemplo, la hesperetina, una flavanona, inhibe la actividad de los genes de la acil-coenzima A:colesterol aciltransferasa y reduce la actividad de la proteína de transferencia de triglicéridos microsomales. También regula al alza el receptor de lipoproteínas de baja densidad . Además, las flavanonas pueden modular las vías de señalización implicadas en la inflamación, la apoptosis y el estrés oxidativo .
Análisis Bioquímico
Biochemical Properties
Flavanone participates in several biochemical reactions, primarily through its interaction with various enzymes and proteins. One key enzyme is flavanone 3-hydroxylase, which catalyzes the hydroxylation of flavanones to dihydroflavonols, a crucial step in the biosynthesis of other flavonoids . Flavanone also interacts with cytochrome P450 enzymes, which are involved in its metabolism and modification. These interactions often involve the transfer of electrons and the formation of intermediate compounds, which can further participate in various metabolic pathways.
Cellular Effects
Flavanone exerts multiple effects on different cell types and cellular processes. It has been shown to influence cell signaling pathways, such as the mitogen-activated protein kinase (MAPK) pathway, which plays a role in cell growth and differentiation . Flavanone can also modulate gene expression by interacting with transcription factors and influencing the transcription of specific genes. Additionally, flavanone impacts cellular metabolism by acting as an antioxidant, scavenging reactive oxygen species (ROS), and protecting cells from oxidative stress .
Molecular Mechanism
At the molecular level, flavanone exerts its effects through various mechanisms. It can bind to specific receptors on the cell surface, initiating a cascade of intracellular signaling events. Flavanone also acts as an enzyme inhibitor, particularly inhibiting enzymes involved in inflammation and cancer progression . Furthermore, flavanone can modulate gene expression by interacting with nuclear receptors and transcription factors, leading to changes in the transcription of target genes .
Temporal Effects in Laboratory Settings
In laboratory settings, the effects of flavanone can vary over time. Studies have shown that flavanone is relatively stable under standard laboratory conditions, but it can degrade under extreme pH or temperature . Long-term exposure to flavanone in cell cultures has been associated with sustained antioxidant activity and protection against oxidative stress . Prolonged exposure may also lead to adaptive cellular responses, such as the upregulation of detoxifying enzymes.
Dosage Effects in Animal Models
The effects of flavanone in animal models are dose-dependent. At low doses, flavanone exhibits beneficial effects, such as anti-inflammatory and antioxidant activities . At higher doses, flavanone can exhibit toxic effects, including liver damage and gastrointestinal disturbances . These adverse effects are often associated with the accumulation of flavanone metabolites, which can interfere with normal cellular functions.
Metabolic Pathways
Flavanone is involved in several metabolic pathways, including the phenylpropanoid pathway, which leads to the biosynthesis of various flavonoids . Enzymes such as chalcone synthase and flavanone 3-hydroxylase play crucial roles in these pathways . Flavanone can also influence metabolic flux by modulating the activity of key enzymes and altering the levels of specific metabolites .
Transport and Distribution
Within cells and tissues, flavanone is transported and distributed through various mechanisms. It can be transported by specific transporter proteins, such as multidrug resistance-associated proteins (MRPs) and glutathione S-transferases (GSTs) . These transporters facilitate the movement of flavanone across cellular membranes and its accumulation in specific cellular compartments. Additionally, flavanone can bind to plasma proteins, which aids in its distribution throughout the body .
Subcellular Localization
Flavanone is localized in various subcellular compartments, including the cytoplasm, nucleus, and mitochondria . Its subcellular localization can influence its activity and function. For example, flavanone localized in the nucleus can directly interact with transcription factors and modulate gene expression . Post-translational modifications, such as phosphorylation, can also affect the subcellular localization and activity of flavanone .
Métodos De Preparación
Rutas Sintéticas y Condiciones de Reacción: Las flavanonas se pueden sintetizar mediante varios métodos. Un enfoque común implica la ciclación intramolecular de las 2’-hidroxicalconas. Este método normalmente utiliza la reacción de condensación de Claisen-Schmidt para formar el intermedio 2’-hidroxicalcona, que luego se cicla para producir flavanona . Otro método implica la ciclación oxidativa catalizada por paladio(II) de las 2’-hidroxihidrocalconas. Este proceso es eficiente y versátil, permitiendo la producción de diversas flavanonas en condiciones suaves .
Métodos de Producción Industrial: La producción industrial de flavanonas a menudo implica la extracción de fuentes naturales, como los cítricos. El proceso de extracción normalmente incluye pasos como la extracción con disolventes, la purificación y la cristalización para obtener flavanonas de alta pureza. También se emplean métodos de extracción asistida por enzimas para mejorar el rendimiento y la pureza .
Análisis De Reacciones Químicas
Tipos de Reacciones: Las flavanonas sufren diversas reacciones químicas, incluyendo la oxidación, la reducción y la sustitución. Por ejemplo, las flavanonas se pueden oxidar para formar flavonas, una clase de compuestos estrechamente relacionada. Este proceso de oxidación a menudo requiere oxidantes fuertes como el yodo .
Reactivos y Condiciones Comunes:
Oxidación: Yodo u otros oxidantes fuertes.
Reducción: Borohidruro de sodio u otros agentes reductores.
Sustitución: Diversos electrófilos y nucleófilos en condiciones apropiadas.
Productos Principales:
Oxidación: Flavonas.
Reducción: Flavan-4-oles.
Sustitución: Diversas flavanonas sustituidas dependiendo de los reactivos utilizados
Comparación Con Compuestos Similares
Las flavanonas son estructuralmente similares a otros flavonoides, como las flavonas, flavonoles e isoflavonas. son únicas en su enlace saturado C2-C3, que las distingue de las flavonas y flavonoles que tienen un doble enlace en esta posición. Esta diferencia estructural influye en su reactividad química y actividad biológica .
Compuestos Similares:
Flavonas: Difieren por tener un doble enlace entre C2 y C3.
Flavonoles: Similares a las flavonas pero con un grupo hidroxilo en la posición C3.
Isoflavonas: Difieren por tener el grupo fenilo en la posición C3 en lugar de C2
Las flavanonas, con su estructura única y diversas actividades biológicas, siguen siendo un foco importante de investigación en diversos campos científicos.
Propiedades
IUPAC Name |
2-phenyl-2,3-dihydrochromen-4-one | |
---|---|---|
Source | PubChem | |
URL | https://pubchem.ncbi.nlm.nih.gov | |
Description | Data deposited in or computed by PubChem | |
InChI |
InChI=1S/C15H12O2/c16-13-10-15(11-6-2-1-3-7-11)17-14-9-5-4-8-12(13)14/h1-9,15H,10H2 | |
Source | PubChem | |
URL | https://pubchem.ncbi.nlm.nih.gov | |
Description | Data deposited in or computed by PubChem | |
InChI Key |
ZONYXWQDUYMKFB-UHFFFAOYSA-N | |
Source | PubChem | |
URL | https://pubchem.ncbi.nlm.nih.gov | |
Description | Data deposited in or computed by PubChem | |
Canonical SMILES |
C1C(OC2=CC=CC=C2C1=O)C3=CC=CC=C3 | |
Source | PubChem | |
URL | https://pubchem.ncbi.nlm.nih.gov | |
Description | Data deposited in or computed by PubChem | |
Molecular Formula |
C15H12O2 | |
Source | PubChem | |
URL | https://pubchem.ncbi.nlm.nih.gov | |
Description | Data deposited in or computed by PubChem | |
DSSTOX Substance ID |
DTXSID9022318 | |
Record name | Flavanone | |
Source | EPA DSSTox | |
URL | https://comptox.epa.gov/dashboard/DTXSID9022318 | |
Description | DSSTox provides a high quality public chemistry resource for supporting improved predictive toxicology. | |
Molecular Weight |
224.25 g/mol | |
Source | PubChem | |
URL | https://pubchem.ncbi.nlm.nih.gov | |
Description | Data deposited in or computed by PubChem | |
CAS No. |
487-26-3 | |
Record name | (±)-Flavanone | |
Source | CAS Common Chemistry | |
URL | https://commonchemistry.cas.org/detail?cas_rn=487-26-3 | |
Description | CAS Common Chemistry is an open community resource for accessing chemical information. Nearly 500,000 chemical substances from CAS REGISTRY cover areas of community interest, including common and frequently regulated chemicals, and those relevant to high school and undergraduate chemistry classes. This chemical information, curated by our expert scientists, is provided in alignment with our mission as a division of the American Chemical Society. | |
Explanation | The data from CAS Common Chemistry is provided under a CC-BY-NC 4.0 license, unless otherwise stated. | |
Record name | Flavanone | |
Source | ChemIDplus | |
URL | https://pubchem.ncbi.nlm.nih.gov/substance/?source=chemidplus&sourceid=0000487263 | |
Description | ChemIDplus is a free, web search system that provides access to the structure and nomenclature authority files used for the identification of chemical substances cited in National Library of Medicine (NLM) databases, including the TOXNET system. | |
Record name | FLAVANONE | |
Source | DTP/NCI | |
URL | https://dtp.cancer.gov/dtpstandard/servlet/dwindex?searchtype=NSC&outputformat=html&searchlist=50393 | |
Description | The NCI Development Therapeutics Program (DTP) provides services and resources to the academic and private-sector research communities worldwide to facilitate the discovery and development of new cancer therapeutic agents. | |
Explanation | Unless otherwise indicated, all text within NCI products is free of copyright and may be reused without our permission. Credit the National Cancer Institute as the source. | |
Record name | 4H-1-Benzopyran-4-one, 2,3-dihydro-2-phenyl- | |
Source | EPA Chemicals under the TSCA | |
URL | https://www.epa.gov/chemicals-under-tsca | |
Description | EPA Chemicals under the Toxic Substances Control Act (TSCA) collection contains information on chemicals and their regulations under TSCA, including non-confidential content from the TSCA Chemical Substance Inventory and Chemical Data Reporting. | |
Record name | Flavanone | |
Source | EPA DSSTox | |
URL | https://comptox.epa.gov/dashboard/DTXSID9022318 | |
Description | DSSTox provides a high quality public chemistry resource for supporting improved predictive toxicology. | |
Record name | 2,3-dihydroflavone | |
Source | European Chemicals Agency (ECHA) | |
URL | https://echa.europa.eu/substance-information/-/substanceinfo/100.006.959 | |
Description | The European Chemicals Agency (ECHA) is an agency of the European Union which is the driving force among regulatory authorities in implementing the EU's groundbreaking chemicals legislation for the benefit of human health and the environment as well as for innovation and competitiveness. | |
Explanation | Use of the information, documents and data from the ECHA website is subject to the terms and conditions of this Legal Notice, and subject to other binding limitations provided for under applicable law, the information, documents and data made available on the ECHA website may be reproduced, distributed and/or used, totally or in part, for non-commercial purposes provided that ECHA is acknowledged as the source: "Source: European Chemicals Agency, http://echa.europa.eu/". Such acknowledgement must be included in each copy of the material. ECHA permits and encourages organisations and individuals to create links to the ECHA website under the following cumulative conditions: Links can only be made to webpages that provide a link to the Legal Notice page. | |
Record name | FLAVANONE | |
Source | FDA Global Substance Registration System (GSRS) | |
URL | https://gsrs.ncats.nih.gov/ginas/app/beta/substances/WX22P730FB | |
Description | The FDA Global Substance Registration System (GSRS) enables the efficient and accurate exchange of information on what substances are in regulated products. Instead of relying on names, which vary across regulatory domains, countries, and regions, the GSRS knowledge base makes it possible for substances to be defined by standardized, scientific descriptions. | |
Explanation | Unless otherwise noted, the contents of the FDA website (www.fda.gov), both text and graphics, are not copyrighted. They are in the public domain and may be republished, reprinted and otherwise used freely by anyone without the need to obtain permission from FDA. Credit to the U.S. Food and Drug Administration as the source is appreciated but not required. | |
Synthesis routes and methods I
Procedure details
Synthesis routes and methods II
Procedure details
Synthesis routes and methods III
Procedure details
Synthesis routes and methods IV
Procedure details
Synthesis routes and methods V
Procedure details
Retrosynthesis Analysis
AI-Powered Synthesis Planning: Our tool employs the Template_relevance Pistachio, Template_relevance Bkms_metabolic, Template_relevance Pistachio_ringbreaker, Template_relevance Reaxys, Template_relevance Reaxys_biocatalysis model, leveraging a vast database of chemical reactions to predict feasible synthetic routes.
One-Step Synthesis Focus: Specifically designed for one-step synthesis, it provides concise and direct routes for your target compounds, streamlining the synthesis process.
Accurate Predictions: Utilizing the extensive PISTACHIO, BKMS_METABOLIC, PISTACHIO_RINGBREAKER, REAXYS, REAXYS_BIOCATALYSIS database, our tool offers high-accuracy predictions, reflecting the latest in chemical research and data.
Strategy Settings
Precursor scoring | Relevance Heuristic |
---|---|
Min. plausibility | 0.01 |
Model | Template_relevance |
Template Set | Pistachio/Bkms_metabolic/Pistachio_ringbreaker/Reaxys/Reaxys_biocatalysis |
Top-N result to add to graph | 6 |
Feasible Synthetic Routes
Q1: What is the basic chemical structure of flavanones?
A1: Flavanones belong to the flavonoid family and are chemically characterized by a diphenylpropane structure (C6-C3-C6) forming two benzene rings (A and B) linked by a three-carbon chain that forms a closed pyran ring (C) with a ketone group at position 4.
Q2: Can you provide the molecular formula and weight of a specific flavanone?
A2: Let's take the example of naringenin, a common flavanone found in citrus fruits. Its molecular formula is C15H12O5, and its molecular weight is 272.25 g/mol.
Q3: How can you differentiate between various flavanone derivatives using spectroscopic techniques?
A3: Spectroscopic methods are crucial for structural elucidation. Nuclear magnetic resonance (NMR) spectroscopy can identify the specific hydrogen and carbon environments, while mass spectrometry (MS) provides the molecular weight and fragmentation patterns. Additionally, UV-Vis spectroscopy helps characterize the electronic transitions related to the chromophore systems within the flavanone structure [, , , , , ].
Q4: How do flavanones interact with biological targets?
A4: Flavanones exert their biological effects through various mechanisms. They have been shown to interact with enzymes like 11β-hydroxysteroid dehydrogenase type 1 (11β-HSD1), inhibiting its reductase activity []. They can also modulate gene expression, influencing pathways related to inflammation, cell interactions, and vascular function []. Additionally, flavanones have demonstrated antioxidant properties by scavenging free radicals and inhibiting oxidative stress [, , , ].
Q5: What are the downstream effects of flavanone interactions with their targets?
A5: The downstream effects of flavanone interactions are diverse and depend on the specific target and biological context. For example, inhibiting 11β-HSD1 reductase activity can impact glucocorticoid metabolism and potentially benefit diabetes management []. Modulating gene expression can affect cellular processes like inflammation and vascular function, potentially contributing to cardiovascular health []. Additionally, their antioxidant properties can protect against cellular damage caused by oxidative stress [, , , ].
Q6: Have any studies investigated the interaction of flavanones with DNA?
A6: Yes, research suggests that certain flavanones, such as 6-hydroxyflavanone, exhibit weak interactions with DNA []. This interaction could be relevant for understanding their potential protective effects against DNA damage caused by oxidative stress.
Q7: How do structural modifications of flavanones influence their biological activity?
A7: Structural modifications significantly impact flavanone activity. Studies have shown that the presence and position of hydroxyl groups, prenyl groups, and glycosylation can alter their cytotoxicity, antioxidant potential, and enzyme inhibitory activity [, , , , ]. For example, the addition of a lavandulyl and hydroxyl group to the flavanone structure significantly enhances cytotoxic activity against mouse macrophage-like Raw 264.7 cells []. Similarly, the presence of a 6-methoxy group in flavanones contributes to their ability to inhibit the bitter taste receptor hTAS2R39 [].
Q8: Are there specific structural features of flavanones that contribute to their bitterness?
A8: Yes, research using bitter taste receptor assays has identified specific structural features contributing to the bitterness of flavanones. The presence of three hydroxyl substitutions on the A and B rings, along with specific hydrogen bond donor and acceptor sites and hydrophobic aromatic rings, are associated with activation of bitter taste receptors like hTAS2R14 and hTAS2R39 [].
Q9: What is known about the metabolism of flavanones in humans?
A9: Flavanones undergo extensive metabolism in the body, primarily in the liver and intestines. They are metabolized into glucuronide and sulfate conjugates, which are then excreted in urine. Studies have shown significant interindividual variability in flavanone bioavailability and metabolism, likely influenced by factors such as genetics, gut microbiota composition, and dietary habits [, , ]. For instance, genetic polymorphisms in genes encoding sulfotransferases (SULT1A1 and SULT1C4) and the ABCC2 transporter are associated with variations in the excretion of phase II flavanone metabolites after orange juice consumption [].
Q10: Can urine flavanone concentrations be used as reliable biomarkers of dietary intake?
A10: While urinary excretion of flavanone metabolites is used to assess flavanone intake, research suggests that urine concentrations may not be ideal biomarkers due to the significant variability in bioavailability and metabolism []. Factors like dose-dependent renal clearance further complicate the interpretation of urinary flavanone levels [].
Q11: How do factors like food processing and the presence of other dietary components influence flavanone bioavailability?
A11: Technological treatments applied to food products, such as high-pressure homogenization, can affect flavanone solubility and particle size, impacting their absorption []. Moreover, consuming flavanones with other dietary components like oat β-glucan can significantly reduce their bioavailability []. For example, ingesting orange juice with 6 g of β-glucan significantly reduced the urinary excretion of flavanone metabolites compared to consuming orange juice alone, suggesting reduced absorption [].
Q12: What are the potential applications of flavanones in various fields?
A12: Flavanones hold promise for applications in diverse fields due to their wide range of biological activities. Their antioxidant and anti-inflammatory properties make them attractive candidates for developing functional foods and nutraceuticals [, , , ]. They also show potential as antimicrobial agents, particularly against Gram-positive bacteria, which could be valuable for food preservation and pharmaceutical development [, ]. Furthermore, research suggests potential applications in managing metabolic disorders like diabetes and cardiovascular diseases [, , , ].
Q13: What are some future research directions for exploring the therapeutic potential of flavanones?
A13: Further research is necessary to fully understand the therapeutic potential of flavanones. Conducting well-designed clinical trials with standardized flavanone doses and formulations is crucial to confirm their efficacy and safety in humans [, ]. Investigating the impact of interindividual variability in metabolism and bioavailability on therapeutic outcomes is also crucial for developing personalized dietary recommendations [, ]. Furthermore, exploring the synergistic effects of flavanones with other bioactive compounds could lead to novel therapeutic strategies [].
Descargo de responsabilidad e información sobre productos de investigación in vitro
Tenga en cuenta que todos los artículos e información de productos presentados en BenchChem están destinados únicamente con fines informativos. Los productos disponibles para la compra en BenchChem están diseñados específicamente para estudios in vitro, que se realizan fuera de organismos vivos. Los estudios in vitro, derivados del término latino "in vidrio", involucran experimentos realizados en entornos de laboratorio controlados utilizando células o tejidos. Es importante tener en cuenta que estos productos no se clasifican como medicamentos y no han recibido la aprobación de la FDA para la prevención, tratamiento o cura de ninguna condición médica, dolencia o enfermedad. Debemos enfatizar que cualquier forma de introducción corporal de estos productos en humanos o animales está estrictamente prohibida por ley. Es esencial adherirse a estas pautas para garantizar el cumplimiento de los estándares legales y éticos en la investigación y experimentación.