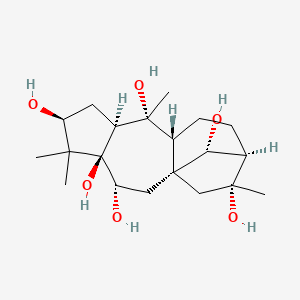
grayanotoxin III
Descripción general
Descripción
Grayanotoxin III is a neurotoxin found in plants of the Ericaceae family, particularly in the Rhododendron genus . It is one of the principal toxic isoforms of grayanotoxins, which are polyhydroxylated cyclic diterpenes characterized by a 5/7/6/5 ring system . Grayanotoxins are known for their ability to interfere with voltage-gated sodium channels, leading to various toxic effects .
Aplicaciones Científicas De Investigación
Grayanotoxin III has several scientific research applications:
Chemistry: Used as a model compound for studying complex diterpenoid synthesis.
Biology: Investigated for its effects on sodium channels in neurons.
Medicine: Studied for its toxic effects and potential therapeutic applications in controlled doses.
Industry: Limited applications due to its toxicity, but used in research settings for studying natural toxins.
Mecanismo De Acción
Target of Action
Grayanotoxin III, also known as Deacylasebotoxin I, is a neurotoxin that primarily targets voltage-gated sodium channels located in the cell membrane of neurons . These channels play a crucial role in the generation and propagation of action potentials in neurons, which are essential for the transmission of signals in the nervous system .
Biochemical Pathways
The primary biochemical pathway affected by this compound is the sodium-potassium pump mechanism, which is responsible for maintaining the resting potential of neurons . By interfering with the function of sodium channels, this compound disrupts the balance of sodium and potassium ions across the neuronal membrane. This disruption leads to a prolonged depolarization phase and delayed repolarization, which can result in continuous firing of action potentials .
Pharmacokinetics
It is known that grayanotoxins are low molecular weight hydrophobic compounds , suggesting that they may be readily absorbed and distributed in the body
Result of Action
The primary result of this compound action is the induction of a state of neuronal hyperexcitability due to prolonged depolarization . This can lead to various symptoms such as muscle twitching, seizures, and even cardiac arrhythmias . The toxin can also cause a slowed heartbeat and a drop in blood pressure .
Action Environment
The action of this compound can be influenced by various environmental factors. For instance, the toxin is produced by plants in the family Ericaceae, specifically members of the genera Rhododendron, among others . These plants grow in various parts of the world, including Europe, North America, Japan, Nepal, and Turkey, and can grow at a variety of altitudes . The concentration of grayanotoxins in these plants can vary depending on environmental conditions, which can influence the toxin’s action, efficacy, and stability .
Safety and Hazards
Análisis Bioquímico
Biochemical Properties
Grayanotoxin III plays a significant role in biochemical reactions by binding to the group II receptor site of voltage-dependent sodium channels . This binding enhances channel activity, leading to prolonged depolarization of the affected cells . The compound interacts with various enzymes, proteins, and other biomolecules, including sodium channels, which are crucial for its neurotoxic effects . The nature of these interactions involves the stabilization of the open state of sodium channels, preventing their inactivation and leading to continuous nerve firing .
Cellular Effects
This compound has profound effects on various types of cells and cellular processes. It influences cell function by altering cell signaling pathways, gene expression, and cellular metabolism . The compound’s interaction with sodium channels leads to prolonged depolarization, which can disrupt normal cellular activities and cause cell death . Additionally, this compound has been shown to affect hormone levels, such as testosterone, luteinizing hormone, and follicle-stimulating hormone, in animal models .
Molecular Mechanism
The molecular mechanism of this compound involves its binding to voltage-dependent sodium channels, which prevents their inactivation and leads to continuous nerve firing . This prolonged depolarization can result in various toxic effects, including muscle weakness, respiratory distress, and cardiac arrhythmias . The compound’s ability to stabilize the open state of sodium channels is a key factor in its neurotoxic effects .
Temporal Effects in Laboratory Settings
In laboratory settings, the effects of this compound can change over time. The compound’s stability and degradation are important factors that influence its long-term effects on cellular function . Studies have shown that this compound can cause acute and chronic effects, with dose-dependent variations in its impact on cellular processes . For example, acute doses of this compound have been found to lower the Johnsen score and decrease the diameter of seminiferous tubules in testicular tissue .
Dosage Effects in Animal Models
The effects of this compound vary with different dosages in animal models. At low doses, the compound may have beneficial effects, such as enhancing sexual performance . At high doses, this compound can cause toxic effects, including hemorrhage, epithelial shedding, and vacuolization in testicular tissue . The threshold effects observed in these studies highlight the importance of dose-dependent variations in the compound’s impact on biological systems .
Metabolic Pathways
This compound is involved in various metabolic pathways, interacting with enzymes and cofactors that influence its activity . The compound’s effects on metabolic flux and metabolite levels are important factors in its overall impact on cellular function . Studies have shown that this compound can affect hormone levels and other metabolic processes in animal models .
Transport and Distribution
The transport and distribution of this compound within cells and tissues are crucial for its biological effects . The compound interacts with transporters and binding proteins that influence its localization and accumulation in specific tissues . These interactions play a key role in the compound’s overall impact on cellular function and its toxic effects .
Subcellular Localization
This compound’s subcellular localization is important for its activity and function . The compound may be directed to specific compartments or organelles through targeting signals or post-translational modifications . These factors influence the compound’s overall impact on cellular processes and its neurotoxic effects .
Métodos De Preparación
Synthetic Routes and Reaction Conditions
The total synthesis of grayanotoxin III has been achieved through various methods. One notable method involves the enantioselective conjugate addition of a compound to 3-methyl cyclohexenone, followed by exposure to iodine chloride and coupling with an organozinc reagent . This process includes several steps such as cyclization, oxidation, and epoxide formation to construct the desired carbon skeleton .
Industrial Production Methods
Industrial production methods for this compound are not well-documented, likely due to its toxic nature and limited commercial applications. Most syntheses are performed in research settings for scientific studies.
Análisis De Reacciones Químicas
Types of Reactions
Grayanotoxin III undergoes various chemical reactions, including:
Oxidation: Conversion of enone to enol ether, followed by further oxidation to form epoxides.
Reduction: Enantioselective reduction of diketones.
Substitution: Coupling reactions with organozinc reagents.
Common Reagents and Conditions
Oxidizing Agents: Iodine chloride, singlet oxygen.
Reducing Agents: Enantioselective reduction reagents.
Coupling Reagents: Organozinc reagents.
Major Products
The major products formed from these reactions include various intermediates leading to the final structure of this compound, such as epoxides and enol ethers .
Comparación Con Compuestos Similares
Grayanotoxin III is part of a group of closely related neurotoxins, including:
Grayanotoxin I: Similar structure but with an acetate group at position 14.
Grayanotoxin II: Lacks the hydroxyl group at position 10.
Grayanotoxin IV: Contains an acetate group at position 10.
This compound is unique due to its specific hydroxylation pattern, which contributes to its distinct toxicological profile .
Propiedades
Número CAS |
4678-45-9 |
---|---|
Fórmula molecular |
C20H34O6 |
Peso molecular |
370.5 g/mol |
Nombre IUPAC |
(1S,3S,4R,6S,8S,9R,10R,13R,14R,16R)-5,5,9,14-tetramethyltetracyclo[11.2.1.01,10.04,8]hexadecane-3,4,6,9,14,16-hexol |
InChI |
InChI=1S/C20H34O6/c1-16(2)13(21)7-12-18(4,25)11-6-5-10-15(23)19(11,9-17(10,3)24)8-14(22)20(12,16)26/h10-15,21-26H,5-9H2,1-4H3/t10-,11+,12+,13+,14+,15-,17-,18-,19+,20+/m1/s1 |
Clave InChI |
BWMFRQKICHXLSH-SGJBMYIASA-N |
SMILES isomérico |
C[C@]1(C[C@@]23C[C@@H]([C@]4([C@@H](C[C@@H](C4(C)C)O)[C@]([C@@H]2CC[C@@H]1[C@H]3O)(C)O)O)O)O |
SMILES |
CC1(C(CC2C1(C(CC34CC(C(C3O)CCC4C2(C)O)(C)O)O)O)O)C |
SMILES canónico |
CC1(C(CC2C1(C(CC34CC(C(C3O)CCC4C2(C)O)(C)O)O)O)O)C |
Apariencia |
Solid powder |
Pictogramas |
Acute Toxic; Irritant |
Pureza |
>98% (or refer to the Certificate of Analysis) |
Vida útil |
>3 years if stored properly |
Solubilidad |
Soluble in DMSO |
Almacenamiento |
Dry, dark and at 0 - 4 C for short term (days to weeks) or -20 C for long term (months to years). |
Sinónimos |
Grayanotoxin III; Deacetylandromedotoxin; Deacylasebotoxin I; Andromedol |
Origen del producto |
United States |
Retrosynthesis Analysis
AI-Powered Synthesis Planning: Our tool employs the Template_relevance Pistachio, Template_relevance Bkms_metabolic, Template_relevance Pistachio_ringbreaker, Template_relevance Reaxys, Template_relevance Reaxys_biocatalysis model, leveraging a vast database of chemical reactions to predict feasible synthetic routes.
One-Step Synthesis Focus: Specifically designed for one-step synthesis, it provides concise and direct routes for your target compounds, streamlining the synthesis process.
Accurate Predictions: Utilizing the extensive PISTACHIO, BKMS_METABOLIC, PISTACHIO_RINGBREAKER, REAXYS, REAXYS_BIOCATALYSIS database, our tool offers high-accuracy predictions, reflecting the latest in chemical research and data.
Strategy Settings
Precursor scoring | Relevance Heuristic |
---|---|
Min. plausibility | 0.01 |
Model | Template_relevance |
Template Set | Pistachio/Bkms_metabolic/Pistachio_ringbreaker/Reaxys/Reaxys_biocatalysis |
Top-N result to add to graph | 6 |
Feasible Synthetic Routes
A: GTX3 primarily binds to voltage-gated sodium channels (VGSCs) [, , , ].
A: GTX3 acts by preventing the inactivation of VGSCs, essentially locking them in an open state. This leads to prolonged sodium influx into cells [, , ].
A: The sustained neuronal excitation caused by GTX3 can result in various symptoms, including bradycardia, hypotension, dizziness, nausea, and in severe cases, seizures and cardiac arrest [, , , ].
A: GTX3 has a molecular formula of C20H32O5 and a molecular weight of 348.45 g/mol [, ].
A: GTX3 is often characterized using NMR (including 2D-NMR techniques like COSY, HMQC, and HMBC), mass spectrometry (MS), and infrared spectroscopy (IR) [, , , , ].
A: Yes, molecular mechanics calculations (MMC) have been used to investigate the conformational preferences of GTX3 and its derivatives. This has provided insights into structure-activity relationships and potential reaction mechanisms [, ].
A: While the provided research doesn't explicitly mention QSAR models for GTX3, the relationship between the compound's structure and its biological activity has been explored, particularly regarding its interaction with sodium channels [, , , ].
A: Research suggests that specific hydroxyl groups and the C10 stereochemistry of GTX3 are crucial for its toxicity. For example, 10-epi-GTX3, a derivative with an altered configuration at C10, exhibits reduced toxicity compared to natural GTX3 [, ].
A: Yes, studies on the acetylation of GTX3 show that the reactivity of hydroxyl groups varies, with the hydroxyl group at position 6 being the most reactive. The different acetylated derivatives may exhibit altered biological activity [].
A: Yes, a study investigated the toxicokinetics of GTX3 in rats after oral administration of mad honey and pure GTX3. The study successfully quantified GTX3 levels in rat blood over time, providing valuable insights into its absorption and elimination [].
A: GTX3's effects have been studied in various cell lines, including rat glioma cells (F98), H9c2 cardiomyoblasts, and Cos-7 cells. These studies have investigated its cytotoxic and antiproliferative properties [, ].
A: Rats and mice are common animal models used in GTX3 toxicity studies. These studies have helped elucidate the compound's effects on the cardiovascular, nervous, and immune systems [, , , , , ].
A: GTX3 can cause a range of symptoms, including nausea, vomiting, dizziness, low blood pressure, bradycardia, and cardiac arrhythmias. In severe cases, it can lead to seizures, respiratory failure, and even death [, , , , , ].
A: While the provided research primarily focuses on acute toxicity, some studies suggest potential long-term effects on the heart, as evidenced by histological changes and inflammatory marker expression in rat heart tissue following chronic GTX3 administration [].
A: The lethal dose of GTX3 varies depending on the species and route of administration. In rats, the lethal dose has been reported to be around 50 μg/kg body weight when administered intravenously [].
A: GTX3 was first isolated and characterized from plants of the Ericaceae family, particularly Rhododendron species, which have been known for their toxicity for centuries [, , , , , ].
ANone: Key milestones include:
- Isolation and structural elucidation: The isolation and characterization of GTX3 and its analogs from natural sources [, , , , ].
- Target identification: The discovery that GTX3 targets voltage-gated sodium channels, leading to a deeper understanding of its mechanism of action [, , , ].
- Structure-activity relationship studies: Investigations into how modifications to the GTX3 structure affect its toxicity, providing insights for potential drug development [, , ].
- Development of analytical methods: The establishment of sensitive and specific methods for detecting and quantifying GTX3 in various matrices, such as honey, blood, and plant material [, , , , , ].
ANone: GTX3 research involves:
- Chemistry: Isolation, structural determination, and chemical synthesis of GTX3 and its derivatives [, , , , , ].
- Biology: Understanding GTX3's interactions with VGSCs, its cellular effects, and its toxicity mechanisms [, , , , , ].
- Pharmacology and Toxicology: Investigating the absorption, distribution, metabolism, excretion, and toxicity of GTX3 in animal models and humans [, , , , ].
- Food Science: Developing methods to detect and quantify GTX3 in honey to ensure consumer safety [, , , , ].
Descargo de responsabilidad e información sobre productos de investigación in vitro
Tenga en cuenta que todos los artículos e información de productos presentados en BenchChem están destinados únicamente con fines informativos. Los productos disponibles para la compra en BenchChem están diseñados específicamente para estudios in vitro, que se realizan fuera de organismos vivos. Los estudios in vitro, derivados del término latino "in vidrio", involucran experimentos realizados en entornos de laboratorio controlados utilizando células o tejidos. Es importante tener en cuenta que estos productos no se clasifican como medicamentos y no han recibido la aprobación de la FDA para la prevención, tratamiento o cura de ninguna condición médica, dolencia o enfermedad. Debemos enfatizar que cualquier forma de introducción corporal de estos productos en humanos o animales está estrictamente prohibida por ley. Es esencial adherirse a estas pautas para garantizar el cumplimiento de los estándares legales y éticos en la investigación y experimentación.