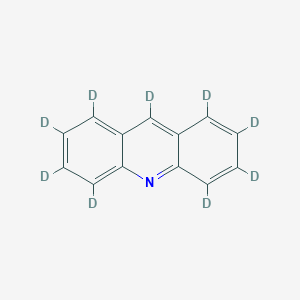
Acridina (D9)
Descripción general
Descripción
Acridine is an organic compound and a nitrogen heterocycle with the formula C₁₃H₉N. It is a planar molecule structurally related to anthracene, with one of the central CH groups replaced by nitrogen. Acridine is known for its broad range of pharmaceutical properties, including anti-inflammatory, anticancer, antimicrobial, antitubercular, antiparasitic, antimalarial, antiviral, and fungicidal activities . Historically, acridine derivatives were used industrially as pigments and dyes .
Aplicaciones Científicas De Investigación
Acridine and its derivatives have numerous scientific research applications:
Mecanismo De Acción
Target of Action
Acridine (D9) primarily targets DNA and acetylcholinesterase (AChE) . The unique planar ring structure of acridine allows it to act as a DNA intercalator . It also shows binding with the catalytic active site (CAS) and the peripheral anionic site (PAS) of AChE .
Mode of Action
Acridine (D9) interacts with its targets primarily through intercalation . This process involves the insertion of the planar acridine molecule between the base pairs of the DNA double helix . This interaction is facilitated by charge transfer and π-stacking interactions, which eventually cause the helical structure of DNA to unwind .
Biochemical Pathways
The intercalation of acridine into DNA affects various biological processes involving DNA and related enzymes . It can inhibit topoisomerase or telomerase enzymes, which play crucial roles in DNA replication and repair . This disruption of normal DNA processes can lead to cell death, particularly in cancer cells .
Pharmacokinetics
The absorption, distribution, metabolism, and excretion (ADME) properties of acridine (D9) are critical for its bioavailability . Acridine (D9) has been shown to penetrate the blood-brain barrier, making it potentially useful for treating CNS tumors or neurodegenerative diseases . The bioavailability of acridine (D9) was calculated to be 83.8% .
Result of Action
The interaction of acridine (D9) with its targets leads to various molecular and cellular effects. Its anticancer activity is particularly notable, with many acridine derivatives having been synthesized and tested for antitumor activity . Normal cells, such as human lymphocytes and Chinese hamster V79 cells, were found to be more susceptible to acridine (D9) than certain human cancer cell lines .
Action Environment
The action, efficacy, and stability of acridine (D9) can be influenced by environmental factors . For instance, the reductive environment in tumor cells can lead to the formation of partially hydrogenated acridines . Moreover, the compound’s action may be affected by its interaction with other bioactive substances, such as DNA repair protein inhibitors .
Safety and Hazards
Direcciones Futuras
More emphasis may be placed on the development of aza-acridine and other heteroatom-substituted acridine derivatives, as well as their synthesis procedures, in order to vary the type and position of the substituent on the acridine core and broaden the applicability of acridine derivatives . The development of acridine derivatives with enhanced therapeutic potency and selectivity as well as luminous materials is the main topic of this review with a focus on recently designed acridine derivatives .
Análisis Bioquímico
Biochemical Properties
Acridine (D9) derivatives are known for their unique physical and chemical properties, biological activities, and industrial applications . They interact with different biomolecular targets due to their semi-planar heterocyclic structure . The unique planar ring structure allows acridine derivatives to act as DNA intercalators and to inhibit topoisomerase or telomerase enzymes .
Cellular Effects
Acridine (D9) and its derivatives have shown high cytotoxic activity . They act against various cell lines such as MDA-MB-231, SK-BR-3, and MCF-7 . The photocytotoxicity of propyl acridine acts against leukemia cell lines . Acridine platinum (Pt) complexes have shown specificity on the evaluated DNA sequences .
Molecular Mechanism
The mode of action of Acridine (D9) is principally due to DNA intercalation . This intercalation, fueled by charge transfer and π-stacking interactions, sandwiches the polyaromatic chromophore between the base pairs of the double helix . This eventually causes the helical structure to unwind .
Temporal Effects in Laboratory Settings
In laboratory settings, Acridine (D9) has shown promising anti-tumor effects against UDP-UGT’s . Over time, the effects of this product may change due to factors such as the product’s stability, degradation, and any long-term effects on cellular function observed in in vitro or in vivo studies .
Dosage Effects in Animal Models
In animal models, the effects of Acridine (D9) vary with different dosages . For instance, several bis(acridine-4-carboxamide) compounds caused adequate growth delays in vivo in the colon 38 tumor models at considerably lower dosages .
Metabolic Pathways
During metabolism, Acridine (D9) is usually converted to more hydrophilic species by oxidation . A formation of practically insoluble acridones can also appear . In tumor cells, the reductive environment can provide partially hydrogenated acridines .
Transport and Distribution
Acridine (D9) is transported and distributed within cells and tissues . Pharmacokinetic data collected at time intervals following a 60 mg/kg oral dose of acridine showed both compounds penetrate the blood–brain barrier yielding peak concentrations .
Subcellular Localization
The subcellular distributions of Acridine (D9) at different drug concentrations were investigated . The NDPI-MS imaging results indicate that Acridine (D9) was accumulated in lysosomes . Additionally, a distinguished subcellular distribution pattern of ethacridine from Acridine (D9) could be visualized .
Métodos De Preparación
Acridine can be synthesized through various methods. One common method involves the cyclization of 2-arylamino benzoic acids using polyphosphoric acid (PPA) to yield acridone derivatives . Another method includes the reaction of dimedone, 4-amino-N-[amino(imino)methyl]-benzenesulfonamide, and 4-cyano-benzaldehyde in the presence of sulfuric acid as a catalyst in water at room temperature . Industrial production often involves the extraction of acridine from coal tar using dilute sulfuric acid, followed by precipitation with potassium dichromate .
Análisis De Reacciones Químicas
Acridine undergoes various chemical reactions, including:
Oxidation: Acridine can be oxidized to acridone using oxidizing agents like potassium permanganate.
Reduction: Reduction of acridine can yield dihydroacridine derivatives.
Substitution: Acridine can undergo electrophilic substitution reactions, such as nitration and halogenation, to form substituted acridines.
Common reagents used in these reactions include sulfuric acid, potassium permanganate, and halogens. Major products formed include acridone, dihydroacridine, and substituted acridines .
Comparación Con Compuestos Similares
Acridine is similar to compounds like acridone, proflavine, acridine orange, and acridine yellow. These compounds share the acridine skeleton but differ in their functional groups and specific applications . For example:
Acridone: Known for its higher stability and use as a DNA intercalator.
Proflavine: Used as an antiseptic and in DNA research.
Acridine Orange: A fluorescent dye used in cell biology.
Acridine Yellow: Used in histology and as a pH indicator.
Acridine’s unique planar structure and broad range of biological activities make it a versatile compound in various fields of research and industry.
Propiedades
IUPAC Name |
1,2,3,4,5,6,7,8,9-nonadeuterioacridine | |
---|---|---|
Source | PubChem | |
URL | https://pubchem.ncbi.nlm.nih.gov | |
Description | Data deposited in or computed by PubChem | |
InChI |
InChI=1S/C13H9N/c1-3-7-12-10(5-1)9-11-6-2-4-8-13(11)14-12/h1-9H/i1D,2D,3D,4D,5D,6D,7D,8D,9D | |
Source | PubChem | |
URL | https://pubchem.ncbi.nlm.nih.gov | |
Description | Data deposited in or computed by PubChem | |
InChI Key |
DZBUGLKDJFMEHC-LOIXRAQWSA-N | |
Source | PubChem | |
URL | https://pubchem.ncbi.nlm.nih.gov | |
Description | Data deposited in or computed by PubChem | |
Canonical SMILES |
C1=CC=C2C(=C1)C=C3C=CC=CC3=N2 | |
Source | PubChem | |
URL | https://pubchem.ncbi.nlm.nih.gov | |
Description | Data deposited in or computed by PubChem | |
Isomeric SMILES |
[2H]C1=C(C(=C2C(=C1[2H])C(=C3C(=C(C(=C(C3=N2)[2H])[2H])[2H])[2H])[2H])[2H])[2H] | |
Source | PubChem | |
URL | https://pubchem.ncbi.nlm.nih.gov | |
Description | Data deposited in or computed by PubChem | |
Molecular Formula |
C13H9N | |
Source | PubChem | |
URL | https://pubchem.ncbi.nlm.nih.gov | |
Description | Data deposited in or computed by PubChem | |
Molecular Weight |
188.27 g/mol | |
Source | PubChem | |
URL | https://pubchem.ncbi.nlm.nih.gov | |
Description | Data deposited in or computed by PubChem | |
Retrosynthesis Analysis
AI-Powered Synthesis Planning: Our tool employs the Template_relevance Pistachio, Template_relevance Bkms_metabolic, Template_relevance Pistachio_ringbreaker, Template_relevance Reaxys, Template_relevance Reaxys_biocatalysis model, leveraging a vast database of chemical reactions to predict feasible synthetic routes.
One-Step Synthesis Focus: Specifically designed for one-step synthesis, it provides concise and direct routes for your target compounds, streamlining the synthesis process.
Accurate Predictions: Utilizing the extensive PISTACHIO, BKMS_METABOLIC, PISTACHIO_RINGBREAKER, REAXYS, REAXYS_BIOCATALYSIS database, our tool offers high-accuracy predictions, reflecting the latest in chemical research and data.
Strategy Settings
Precursor scoring | Relevance Heuristic |
---|---|
Min. plausibility | 0.01 |
Model | Template_relevance |
Template Set | Pistachio/Bkms_metabolic/Pistachio_ringbreaker/Reaxys/Reaxys_biocatalysis |
Top-N result to add to graph | 6 |
Feasible Synthetic Routes
Q1: What makes Acridine-d9 suitable for studying electron spin echo envelope modulation (ESEEM) in zero magnetic field?
A1: Acridine-d9, when embedded in a suitable matrix like fluorene-h10, forms a system where its photoexcited triplet state can be effectively studied using optically detected magnetic resonance (ODMR) [, ]. This technique, coupled with ESEEM, allows for investigating the interaction between the electron spin of the excited acridine-d9 molecule and the surrounding nuclear spins, even in the absence of a strong external magnetic field. The deuteration of Acridine (replacing hydrogens with deuteriums) minimizes the interference from hydrogen nuclei, allowing for a clearer observation of the nitrogen quadrupole splitting in the ESEEM spectrum [].
Q2: What key differences in ESEEM spectra were observed between high and zero magnetic field measurements of Acridine-d9?
A2: The research highlighted qualitative differences in the ESEEM effect observed in the Acridine-d9 triplet state when measured under low-field versus high-field conditions []. These differences stem from the distinct behavior of the total spin angular momentum operator and the hyperfine interaction in these varying magnetic field regimes. At zero field, the total spin angular momentum operator dominates, while at high fields, the hyperfine interaction plays a more significant role.
Descargo de responsabilidad e información sobre productos de investigación in vitro
Tenga en cuenta que todos los artículos e información de productos presentados en BenchChem están destinados únicamente con fines informativos. Los productos disponibles para la compra en BenchChem están diseñados específicamente para estudios in vitro, que se realizan fuera de organismos vivos. Los estudios in vitro, derivados del término latino "in vidrio", involucran experimentos realizados en entornos de laboratorio controlados utilizando células o tejidos. Es importante tener en cuenta que estos productos no se clasifican como medicamentos y no han recibido la aprobación de la FDA para la prevención, tratamiento o cura de ninguna condición médica, dolencia o enfermedad. Debemos enfatizar que cualquier forma de introducción corporal de estos productos en humanos o animales está estrictamente prohibida por ley. Es esencial adherirse a estas pautas para garantizar el cumplimiento de los estándares legales y éticos en la investigación y experimentación.