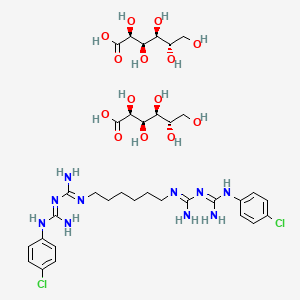
Gluconato de clorhexidina
Descripción general
Descripción
CHLORHEXIDINE DIGLUCONATE is a broad-spectrum antimicrobial agent widely used as a disinfectant and antiseptic. It is effective against a range of microorganisms, including bacteria, yeasts, and viruses. The compound is commonly used in healthcare settings for skin disinfection before surgery, cleaning wounds, and preventing dental plaque. It is also used in various formulations such as solutions, gels, and impregnated medical devices .
Aplicaciones Científicas De Investigación
Antimicrobial Properties
Chlorhexidine digluconate exhibits strong antimicrobial activity against Gram-positive and Gram-negative bacteria, fungi, and some viruses. Its mechanism of action involves disrupting microbial cell membranes, leading to cell lysis and death. This property makes it particularly useful in clinical settings for preventing infections.
Table 1: Antimicrobial Efficacy of Chlorhexidine Digluconate
Microorganism | Minimum Inhibitory Concentration (MIC) |
---|---|
Staphylococcus aureus | 0.5 - 4 mg/L |
Escherichia coli | 4 - 8 mg/L |
Pseudomonas aeruginosa | 2 - 16 mg/L |
Candida albicans | 1 - 4 mg/L |
Dental Applications
In dentistry, chlorhexidine digluconate is commonly used as an oral rinse to reduce plaque formation and gingivitis. It is effective in maintaining oral hygiene, especially in patients with periodontal disease. Studies show that chlorhexidine mouth rinses can significantly decrease the levels of oral pathogens.
Case Study: Efficacy in Periodontal Treatment
A clinical study evaluated the effect of chlorhexidine digluconate mouth rinses on patients undergoing periodontal therapy. Results indicated a significant reduction in probing depth and clinical attachment loss after six months of treatment, highlighting its role in improving periodontal health .
Surgical Applications
Chlorhexidine digluconate is frequently employed as a surgical scrub and skin disinfectant due to its rapid action and residual antimicrobial effects. It is used preoperatively to reduce the risk of surgical site infections.
Case Study: Impact on Surgical Site Infections
A randomized controlled trial assessed the effectiveness of chlorhexidine digluconate versus povidone-iodine for surgical site preparation. The study found that chlorhexidine significantly reduced the incidence of postoperative infections compared to povidone-iodine .
Veterinary Medicine
In veterinary practice, chlorhexidine digluconate is utilized for treating skin infections and as a disinfectant for surgical instruments. Its application in veterinary dermatology has shown promising results in managing conditions like pyoderma.
Case Study: Treatment of Canine Pyoderma
A study investigated the effectiveness of a combined protocol using a 4% chlorhexidine digluconate shampoo and solution for treating superficial pyoderma in dogs. The results demonstrated significant improvement in clinical signs and microbial reduction .
Wound Care
Chlorhexidine digluconate is also used in wound care management due to its ability to prevent infection and promote healing. It can be applied topically or incorporated into wound dressings.
Research Findings on Wound Healing
A study explored the effects of chlorhexidine digluconate on early wound healing in human subjects. Findings suggested that its application led to improved healing rates and reduced bacterial colonization at the wound site .
Mecanismo De Acción
Target of Action
Chlorhexidine gluconate is a broad-spectrum antimicrobial biguanide . Its primary targets are a wide range of pathogens, including Gram-positive bacteria, Gram-negative bacteria, yeasts, and viruses . It acts on the cell walls and extramicrobial complexes of these microorganisms .
Mode of Action
The mode of action of chlorhexidine gluconate involves its interaction with the negatively charged microbial cell surfaces . The positively charged chlorhexidine molecule reacts with these surfaces, destroying the integrity of the cell and allowing leakage of intracellular material . This reaction also enables chlorhexidine to enter the cell, causing precipitation of cytoplasmic components and ultimately leading to cell death .
Biochemical Pathways
Chlorhexidine gluconate affects several biochemical pathways. It disrupts the cell membrane, leading to leakage of components and cell death . In low concentrations, it affects the integrity of the cell wall . Once the cell wall is damaged, chlorhexidine crosses into the cell itself and attacks the cytoplasmic membrane . In high concentrations, chlorhexidine causes the cytoplasm to congeal or solidify .
Pharmacokinetics
Chlorhexidine gluconate exhibits unique pharmacokinetic properties. Approximately 30% of the active ingredient is retained in the oral cavity following rinsing and is slowly released into oral fluids . It is very poorly absorbed from the gastrointestinal tract .
Result of Action
The result of chlorhexidine gluconate’s action is the effective elimination of a broad range of pathogens. Upon application in vitro, chlorhexidine can kill nearly 100% of Gram-positive and Gram-negative bacteria within 30 seconds .
Action Environment
Protein-bound chlorhexidine releases slowly, leading to prolonged activity . This property makes chlorhexidine gluconate an effective antiseptic in various healthcare applications .
Análisis Bioquímico
Biochemical Properties
Chlorhexidine gluconate plays a significant role in biochemical reactions due to its ability to disrupt microbial cell membranes. It interacts with various biomolecules, including enzymes and proteins, by binding to negatively charged phosphate groups on microbial cell surfaces. This interaction destabilizes the cell membrane, leading to leakage of intracellular contents and ultimately cell death . Additionally, chlorhexidine gluconate can precipitate cytoplasmic components at higher concentrations, enhancing its bactericidal effects .
Cellular Effects
Chlorhexidine gluconate exerts profound effects on various cell types and cellular processes. It disrupts the integrity of microbial cell membranes, causing leakage of intracellular substances such as potassium and phosphorous . This disruption affects cell signaling pathways and gene expression, leading to altered cellular metabolism and cell death. In higher concentrations, chlorhexidine gluconate can cause cytoplasmic precipitation, further contributing to its antimicrobial activity .
Molecular Mechanism
The molecular mechanism of chlorhexidine gluconate involves its cationic nature, which allows it to bind to negatively charged bacterial cell walls. This binding disrupts the cell membrane’s integrity, leading to osmotic imbalance and leakage of intracellular contents . At higher concentrations, chlorhexidine gluconate causes cytoplasmic components to precipitate, resulting in cell death . Additionally, it can generate reactive oxygen species (ROS), leading to lipid peroxidation and further membrane damage .
Temporal Effects in Laboratory Settings
In laboratory settings, the effects of chlorhexidine gluconate can vary over time. Its stability and degradation depend on factors such as concentration and environmental conditions. Studies have shown that chlorhexidine gluconate maintains its antimicrobial activity over extended periods, although its effectiveness may decrease with prolonged exposure . Long-term effects on cellular function include potential toxicity to proliferating skin cells, which may impact wound healing .
Dosage Effects in Animal Models
The effects of chlorhexidine gluconate vary with different dosages in animal models. At lower concentrations, it exhibits bacteriostatic properties, inhibiting bacterial growth without causing cell death . Higher concentrations result in bactericidal effects, leading to cell death and potential toxicity. Studies have shown that excessive doses of chlorhexidine gluconate can cause adverse effects such as gastrointestinal issues and rashes in animals . It is crucial to determine the appropriate dosage to balance efficacy and safety.
Metabolic Pathways
Chlorhexidine gluconate is poorly absorbed in the gastrointestinal tract, limiting its metabolic conversion . As a result, it primarily exerts its effects locally at the site of application. The compound’s antimicrobial activity is dose-dependent, with higher concentrations exhibiting stronger bactericidal effects . Chlorhexidine gluconate does not undergo significant metabolic transformation, making it a stable and effective antiseptic agent.
Transport and Distribution
Chlorhexidine gluconate is transported and distributed within cells and tissues through its interaction with negatively charged bacterial cell walls . It binds to the cell membrane, causing disruption and leakage of intracellular contents. In oral applications, approximately 30% of chlorhexidine gluconate is retained in the oral cavity and slowly released into oral fluids . Its poor absorption from the gastrointestinal tract limits systemic distribution, ensuring localized antimicrobial activity.
Subcellular Localization
Chlorhexidine gluconate primarily localizes to the cell membrane and cytoplasm of microbial cells . Its cationic nature allows it to bind to negatively charged sites on the cell membrane, leading to membrane disruption and cell death. The compound’s ability to generate reactive oxygen species further contributes to its antimicrobial activity by causing lipid peroxidation and membrane damage . Chlorhexidine gluconate’s subcellular localization is crucial for its effectiveness as an antiseptic agent.
Métodos De Preparación
Synthetic Routes and Reaction Conditions
CHLORHEXIDINE DIGLUCONATE is synthesized through a multi-step process involving the reaction of 1,6-dichlorohexane with 4-chlorophenylbiguanide. The reaction is typically carried out in an organic solvent under controlled temperature and pH conditions. The resulting product is then purified and converted into its gluconate salt form by reacting with gluconic acid .
Industrial Production Methods
Industrial production of chlorhexidine gluconate involves large-scale synthesis using similar reaction conditions as in laboratory synthesis. The process includes the use of high-purity reagents and advanced purification techniques to ensure the final product meets stringent quality standards. The compound is then formulated into various products such as antiseptic solutions, mouthwashes, and wound dressings .
Análisis De Reacciones Químicas
Types of Reactions
CHLORHEXIDINE DIGLUCONATE undergoes several types of chemical reactions, including:
Oxidation: CHLORHEXIDINE DIGLUCONATE can be oxidized under specific conditions, leading to the formation of various oxidation products.
Reduction: The compound can be reduced, although this reaction is less common.
Substitution: CHLORHEXIDINE DIGLUCONATE can undergo substitution reactions, particularly in the presence of strong nucleophiles.
Common Reagents and Conditions
Oxidation: Common oxidizing agents include hydrogen peroxide and potassium permanganate.
Reduction: Reducing agents such as sodium borohydride can be used.
Substitution: Strong nucleophiles like sodium hydroxide or potassium hydroxide are often employed.
Major Products Formed
The major products formed from these reactions depend on the specific conditions and reagents used. For example, oxidation can lead to the formation of chlorhexidine derivatives with altered antimicrobial properties .
Comparación Con Compuestos Similares
Similar Compounds
- Chlorhexidine diacetate
- Chlorhexidine dihydrochloride
- Chlorhexidine dihydrobromide
- Triclosan
- Povidone-iodine
Uniqueness
CHLORHEXIDINE DIGLUCONATE is unique due to its broad-spectrum antimicrobial activity, high efficacy, and stability over a wide pH range. Unlike other antiseptics, it has a prolonged residual effect, making it highly effective in preventing infections over extended periods .
Actividad Biológica
Chlorhexidine digluconate (CHX) is a widely used antiseptic with significant biological activity, particularly in wound management, oral hygiene, and infection control. This article explores its mechanisms of action, effectiveness, cytotoxicity, and clinical applications based on diverse research findings.
Chlorhexidine digluconate possesses both bactericidal and bacteriostatic properties. At higher concentrations (greater than 0.1%), CHX disrupts bacterial cell membranes, leading to cell lysis and death. Conversely, at lower concentrations (0.02–0.06%), it inhibits bacterial growth without killing the cells, effectively preventing colonization on surfaces such as teeth and skin .
- Bactericidal Action : High concentrations cause irreversible damage to the bacterial cell membrane, resulting in coagulation of cytoplasmic components.
- Bacteriostatic Action : Lower concentrations alter the cell membrane's integrity, increasing permeability and causing leakage of intracellular components .
Cytotoxicity
While effective against a range of microorganisms, CHX exhibits cytotoxic effects on human cells, particularly fibroblasts and keratinocytes. In vitro studies have shown that concentrations as low as 0.05% can lead to cell death within 24 hours . Notably:
- Fibroblast Proliferation : Exposure to 0.0032% CHX significantly reduces fibroblast proliferation (p=0.05), while a lower concentration (0.0004%) can enhance it by 16% .
- Wound Healing : A study indicated that CHX promotes a scar wound healing response by increasing collagen deposition and myofibroblast differentiation in gingival tissue after surgical procedures .
Wound Management
CHX is commonly employed in wound care due to its ability to reduce bacterial load. However, evidence suggests that its effectiveness may be limited by its cytotoxicity to fibroblasts necessary for wound healing . Its application in surgical settings has been scrutinized due to reports of adverse events such as anaphylaxis and respiratory distress following exposure or ingestion .
Oral Hygiene
In dentistry, CHX is regarded as the "gold standard" for managing plaque and gingivitis. Rinsing with CHX significantly reduces plaque accumulation by approximately 60% and gingivitis severity by 50-80% after consistent use over several weeks . The sustained release from oral surfaces contributes to its long-lasting effects.
Case Studies
Several case studies highlight the practical applications of CHX:
- Mouth Ulcer Treatment : A study involving pediatric patients demonstrated the efficacy of 2% CHX gauze in alleviating pain associated with oral ulcers .
- Post-Surgical Care : Research on post-surgical patients indicated that CHX mouth rinses not only reduced bacterial load but also improved healing outcomes through enhanced collagen deposition and reduced inflammation markers .
Safety Profile
Despite its benefits, CHX is associated with potential side effects, including skin irritation and ocular toxicity upon exposure . Reports of serious adverse reactions necessitate caution during use:
Propiedades
IUPAC Name |
2-[6-[[amino-[[amino-(4-chloroanilino)methylidene]amino]methylidene]amino]hexyl]-1-[amino-(4-chloroanilino)methylidene]guanidine;2,3,4,5,6-pentahydroxyhexanoic acid | |
---|---|---|
Details | Computed by Lexichem TK 2.7.0 (PubChem release 2021.05.07) | |
Source | PubChem | |
URL | https://pubchem.ncbi.nlm.nih.gov | |
Description | Data deposited in or computed by PubChem | |
InChI |
InChI=1S/C22H30Cl2N10.2C6H12O7/c23-15-5-9-17(10-6-15)31-21(27)33-19(25)29-13-3-1-2-4-14-30-20(26)34-22(28)32-18-11-7-16(24)8-12-18;2*7-1-2(8)3(9)4(10)5(11)6(12)13/h5-12H,1-4,13-14H2,(H5,25,27,29,31,33)(H5,26,28,30,32,34);2*2-5,7-11H,1H2,(H,12,13) | |
Details | Computed by InChI 1.0.6 (PubChem release 2021.05.07) | |
Source | PubChem | |
URL | https://pubchem.ncbi.nlm.nih.gov | |
Description | Data deposited in or computed by PubChem | |
InChI Key |
YZIYKJHYYHPJIB-UHFFFAOYSA-N | |
Details | Computed by InChI 1.0.6 (PubChem release 2021.05.07) | |
Source | PubChem | |
URL | https://pubchem.ncbi.nlm.nih.gov | |
Description | Data deposited in or computed by PubChem | |
Canonical SMILES |
C1=CC(=CC=C1NC(=NC(=NCCCCCCN=C(N)N=C(N)NC2=CC=C(C=C2)Cl)N)N)Cl.C(C(C(C(C(C(=O)O)O)O)O)O)O.C(C(C(C(C(C(=O)O)O)O)O)O)O | |
Details | Computed by OEChem 2.3.0 (PubChem release 2021.05.07) | |
Source | PubChem | |
URL | https://pubchem.ncbi.nlm.nih.gov | |
Description | Data deposited in or computed by PubChem | |
Molecular Formula |
C34H54Cl2N10O14 | |
Details | Computed by PubChem 2.1 (PubChem release 2021.05.07) | |
Source | PubChem | |
URL | https://pubchem.ncbi.nlm.nih.gov | |
Description | Data deposited in or computed by PubChem | |
Molecular Weight |
897.8 g/mol | |
Details | Computed by PubChem 2.1 (PubChem release 2021.05.07) | |
Source | PubChem | |
URL | https://pubchem.ncbi.nlm.nih.gov | |
Description | Data deposited in or computed by PubChem | |
CAS No. |
18472-51-0 | |
Record name | D-Gluconic acid, compd. with N1,N14-bis(4-chlorophenyl)-3,12-diimino-2,4,11,13-tetraazatetradecanediimidamide (2:1) | |
Source | EPA Chemicals under the TSCA | |
URL | https://www.epa.gov/chemicals-under-tsca | |
Description | EPA Chemicals under the Toxic Substances Control Act (TSCA) collection contains information on chemicals and their regulations under TSCA, including non-confidential content from the TSCA Chemical Substance Inventory and Chemical Data Reporting. | |
Record name | D-gluconic acid, compound with N,N''-bis(4-chlorophenyl)-3,12-diimino-2,4,11,13-tetraazatetradecanediamidine (2:1) | |
Source | European Chemicals Agency (ECHA) | |
URL | https://echa.europa.eu/substance-information/-/substanceinfo/100.038.489 | |
Description | The European Chemicals Agency (ECHA) is an agency of the European Union which is the driving force among regulatory authorities in implementing the EU's groundbreaking chemicals legislation for the benefit of human health and the environment as well as for innovation and competitiveness. | |
Explanation | Use of the information, documents and data from the ECHA website is subject to the terms and conditions of this Legal Notice, and subject to other binding limitations provided for under applicable law, the information, documents and data made available on the ECHA website may be reproduced, distributed and/or used, totally or in part, for non-commercial purposes provided that ECHA is acknowledged as the source: "Source: European Chemicals Agency, http://echa.europa.eu/". Such acknowledgement must be included in each copy of the material. ECHA permits and encourages organisations and individuals to create links to the ECHA website under the following cumulative conditions: Links can only be made to webpages that provide a link to the Legal Notice page. | |
Retrosynthesis Analysis
AI-Powered Synthesis Planning: Our tool employs the Template_relevance Pistachio, Template_relevance Bkms_metabolic, Template_relevance Pistachio_ringbreaker, Template_relevance Reaxys, Template_relevance Reaxys_biocatalysis model, leveraging a vast database of chemical reactions to predict feasible synthetic routes.
One-Step Synthesis Focus: Specifically designed for one-step synthesis, it provides concise and direct routes for your target compounds, streamlining the synthesis process.
Accurate Predictions: Utilizing the extensive PISTACHIO, BKMS_METABOLIC, PISTACHIO_RINGBREAKER, REAXYS, REAXYS_BIOCATALYSIS database, our tool offers high-accuracy predictions, reflecting the latest in chemical research and data.
Strategy Settings
Precursor scoring | Relevance Heuristic |
---|---|
Min. plausibility | 0.01 |
Model | Template_relevance |
Template Set | Pistachio/Bkms_metabolic/Pistachio_ringbreaker/Reaxys/Reaxys_biocatalysis |
Top-N result to add to graph | 6 |
Feasible Synthetic Routes
Q1: How does chlorhexidine gluconate exert its antimicrobial effect?
A1: [] Chlorhexidine gluconate disrupts the bacterial cell membrane, leading to leakage of intracellular components and ultimately cell death. This broad-spectrum activity makes it effective against various gram-positive and gram-negative bacteria, as well as fungi.
Q2: What is the molecular formula and weight of chlorhexidine gluconate?
A2: While the provided abstracts do not explicitly state the molecular formula and weight of chlorhexidine gluconate, these details can be readily found in chemical databases like PubChem or ChemSpider.
Q3: Can chlorhexidine gluconate be incorporated into wound dressings?
A4: Yes, [] research has explored incorporating chlorhexidine gluconate into hydrophilic polyurethane foam dressings. Studies demonstrated in vitro antimicrobial activity against a range of microorganisms, including antibiotic-resistant strains of Staphylococcus and Enterococcus species, as well as Candida species.
Q4: Does chlorhexidine gluconate impact the viscoelastic properties of acrylic resins used in dentistry?
A5: Research [] comparing the impact of chlorhexidine gluconate on polymethyl methacrylate (PMMA) and polyoxymethylene (POM) found that while chlorhexidine gluconate did not significantly affect PMMA viscoelasticity, it did lower the storage modulus and glass transition temperature of POM. This suggests material-specific interactions that require careful consideration in dental applications.
Q5: Is chlorhexidine gluconate effective against Enterococcus faecalis in root canal treatment?
A6: Yes, multiple studies [, , , ] confirm the efficacy of chlorhexidine gluconate against Enterococcus faecalis, a bacterium commonly found in persistent root canal infections. Its efficacy has been demonstrated both in vitro and in vivo, with various studies highlighting its superior antibacterial action compared to sodium hypochlorite.
Q6: Can chlorhexidine gluconate be used to prevent alveolar osteitis after tooth extraction?
A7: Research suggests [] that while chlorhexidine gluconate alone might not be sufficient to completely prevent alveolar osteitis (dry socket), combining it with an antibiotic like amoxicillin plus clavulanic acid significantly reduces its incidence after mandibular third molar extractions.
Q7: Are there any concerns regarding the toxicity of chlorhexidine gluconate?
A9: While generally considered safe for topical use, [, , ] chlorhexidine gluconate can cause allergic reactions in some individuals, ranging from mild skin irritation to severe anaphylactic shock. Studies on rat models [, ] also show that chlorhexidine gluconate, when administered intrathecally, can induce inflammatory responses in lung tissues.
Q8: Does chlorhexidine gluconate impact oral cells?
A10: While research [] shows that chlorhexidine gluconate can have cytotoxic effects on oral cell lines, combining it with benzydamine hydrochloride appears to mitigate this toxicity and maintain cell viability. Further research is needed to understand the clinical implications of these findings.
Q9: Are there any natural alternatives to chlorhexidine gluconate for oral health applications?
A11: Several studies explored natural alternatives, like green tea extract (Camellia sinensis) [, , ] and rambai leaf extract (Sonneratia caseolaris) []. While these natural extracts show promise in inhibiting the growth of oral pathogens like Candida albicans and Streptococcus sanguinis, their efficacy compared to chlorhexidine gluconate requires further investigation.
Q10: What analytical methods are commonly employed to study chlorhexidine gluconate?
A12: Researchers utilize a variety of techniques, including agar diffusion assays [, , , ], broth microdilution methods [], and scanning electron microscopy [, ], to evaluate the antimicrobial activity and effects of chlorhexidine gluconate. These methods help determine minimum inhibitory concentrations, assess its impact on bacterial morphology, and evaluate its effectiveness in removing smear layers in dental applications.
Descargo de responsabilidad e información sobre productos de investigación in vitro
Tenga en cuenta que todos los artículos e información de productos presentados en BenchChem están destinados únicamente con fines informativos. Los productos disponibles para la compra en BenchChem están diseñados específicamente para estudios in vitro, que se realizan fuera de organismos vivos. Los estudios in vitro, derivados del término latino "in vidrio", involucran experimentos realizados en entornos de laboratorio controlados utilizando células o tejidos. Es importante tener en cuenta que estos productos no se clasifican como medicamentos y no han recibido la aprobación de la FDA para la prevención, tratamiento o cura de ninguna condición médica, dolencia o enfermedad. Debemos enfatizar que cualquier forma de introducción corporal de estos productos en humanos o animales está estrictamente prohibida por ley. Es esencial adherirse a estas pautas para garantizar el cumplimiento de los estándares legales y éticos en la investigación y experimentación.