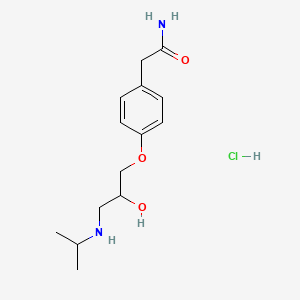
Atenolol hydrochloride
- Haga clic en CONSULTA RÁPIDA para recibir una cotización de nuestro equipo de expertos.
- Con productos de calidad a un precio COMPETITIVO, puede centrarse más en su investigación.
Descripción general
Descripción
Atenolol Hydrochloride is a selective ß1 receptor antagonist.
Aplicaciones Científicas De Investigación
Cardiovascular Impacts : Atenolol's effectiveness in reducing cardiovascular morbidity and mortality in hypertensive patients has been a subject of research. Studies have shown that despite differences in blood pressure lowering, there were no significant outcome differences between atenolol and placebo in terms of all-cause mortality, cardiovascular mortality, or myocardial infarction. However, it did tend to lower the risk of stroke compared to placebo (Carlberg, Samuelsson, & Lindholm, 2004).
Analytical Applications : Atenolol has been the subject of studies focusing on improving chromatographic methods for its determination, particularly in combination with other drugs like hydrochlorothiazide and chlorthalidone. These methods are essential for quality control in pharmaceutical labs (Elkady, Fouad, & Faquih, 2020).
Environmental Impact : Research has also focused on the environmental impact of atenolol, particularly its presence in wastewater and subsequent removal. Studies have explored the efficiency of adsorption processes using materials like granular activated carbon for the removal of atenolol from aqueous solutions, with significant potential for environmental remediation (Haro, Vecchio, Marcilio, & Féris, 2017).
Ecotoxicology Studies : Atenolol's chronic toxicity to aquatic species has been assessed. For instance, studies using fathead minnows have suggested that atenolol has low chronic toxicity to fish, even at environmentally realistic concentrations (Winter et al., 2008).
Synthesis of Impurities : Research on atenolol also includes the synthesis of its impurities, which is crucial for understanding the drug's stability and safety profile (Pai & Patil, 2012).
Degradation Studies : Studies on atenolol have examined its degradation in various conditions, such as in the presence of hydroxyl radicals, which is vital for understanding its environmental persistence and potential impact (Nejumal, Manoj, Aravind, & Aravindakumar, 2014).
Stereoisomer Studies : The synthesis and structural analysis of atenolol's stereoisomers have been a subject of interest, particularly in understanding the drug's stereochemistry and its implications for efficacy and safety (Kandula, Vepuri, Devarajegowda, & Raja, 2018).
Drug Extraction and Separation : Research has been conducted on efficient and selective methods for the separation of atenolol, such as using molecularly imprinted polymer sorbents. This is important for detecting and monitoring the level of atenolol in biological samples like blood serum (Hasanah et al., 2019).
Corrosion Inhibition Studies : Atenolol has been studied as a corrosion inhibitor for mild steel in acidic environments. Understanding the interaction of drugs like atenolol with metals is important for various industrial applications (Karthik & Sundaravadivelu, 2016).
Propiedades
Número CAS |
51706-40-2 |
---|---|
Fórmula molecular |
C14H23ClN2O3 |
Peso molecular |
302.8 g/mol |
Nombre IUPAC |
2-[4-[2-hydroxy-3-(propan-2-ylamino)propoxy]phenyl]acetamide;hydrochloride |
InChI |
InChI=1S/C14H22N2O3.ClH/c1-10(2)16-8-12(17)9-19-13-5-3-11(4-6-13)7-14(15)18;/h3-6,10,12,16-17H,7-9H2,1-2H3,(H2,15,18);1H |
Clave InChI |
FFDDLJYKJQGSPW-UHFFFAOYSA-N |
SMILES |
CC(C)NCC(COC1=CC=C(C=C1)CC(=O)N)O.Cl |
SMILES canónico |
CC(C)NCC(COC1=CC=C(C=C1)CC(=O)N)O.Cl |
Apariencia |
Solid powder |
Otros números CAS |
51706-40-2 |
Pureza |
>98% (or refer to the Certificate of Analysis) |
Vida útil |
>2 years if stored properly |
Solubilidad |
Soluble in DMSO |
Almacenamiento |
Dry, dark and at 0 - 4 C for short term (days to weeks) or -20 C for long term (months to years). |
Sinónimos |
Atenolol Hydrochloride |
Origen del producto |
United States |
Retrosynthesis Analysis
AI-Powered Synthesis Planning: Our tool employs the Template_relevance Pistachio, Template_relevance Bkms_metabolic, Template_relevance Pistachio_ringbreaker, Template_relevance Reaxys, Template_relevance Reaxys_biocatalysis model, leveraging a vast database of chemical reactions to predict feasible synthetic routes.
One-Step Synthesis Focus: Specifically designed for one-step synthesis, it provides concise and direct routes for your target compounds, streamlining the synthesis process.
Accurate Predictions: Utilizing the extensive PISTACHIO, BKMS_METABOLIC, PISTACHIO_RINGBREAKER, REAXYS, REAXYS_BIOCATALYSIS database, our tool offers high-accuracy predictions, reflecting the latest in chemical research and data.
Strategy Settings
Precursor scoring | Relevance Heuristic |
---|---|
Min. plausibility | 0.01 |
Model | Template_relevance |
Template Set | Pistachio/Bkms_metabolic/Pistachio_ringbreaker/Reaxys/Reaxys_biocatalysis |
Top-N result to add to graph | 6 |
Feasible Synthetic Routes
Descargo de responsabilidad e información sobre productos de investigación in vitro
Tenga en cuenta que todos los artículos e información de productos presentados en BenchChem están destinados únicamente con fines informativos. Los productos disponibles para la compra en BenchChem están diseñados específicamente para estudios in vitro, que se realizan fuera de organismos vivos. Los estudios in vitro, derivados del término latino "in vidrio", involucran experimentos realizados en entornos de laboratorio controlados utilizando células o tejidos. Es importante tener en cuenta que estos productos no se clasifican como medicamentos y no han recibido la aprobación de la FDA para la prevención, tratamiento o cura de ninguna condición médica, dolencia o enfermedad. Debemos enfatizar que cualquier forma de introducción corporal de estos productos en humanos o animales está estrictamente prohibida por ley. Es esencial adherirse a estas pautas para garantizar el cumplimiento de los estándares legales y éticos en la investigación y experimentación.