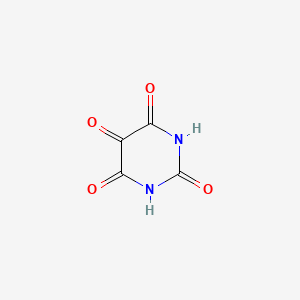
Alloxan
Descripción general
Descripción
Alloxan (2,4,5,6-pyrimidinetetrone) is a pyrimidine derivative first isolated in 1818 and widely used to induce experimental diabetes in animals due to its selective toxicity toward pancreatic β-cells . Structurally, it is a hydrophilic compound with a molar mass of 142.07 g/mol and freely soluble in aqueous solutions as this compound hydrate . Its diabetogenic effect arises from two distinct mechanisms:
Inhibition of glucokinase, the glucose sensor of β-cells, which blocks glucose-stimulated insulin secretion .
Generation of reactive oxygen species (ROS), particularly hydroxyl radicals, via redox cycling with its reduced form, dialuric acid. This induces oxidative stress and selective β-cell necrosis .
This compound is administered intravenously, intraperitoneally, or subcutaneously at doses ranging from 90–200 mg/kg, with 150 mg/kg being the most common . Its rapid uptake by β-cells via the GLUT2 glucose transporter underpins its cellular specificity .
Métodos De Preparación
Chemical Synthesis and Stability Challenges
Alloxan is traditionally synthesized via the oxidation of uric acid, though detailed synthetic protocols are sparingly documented in modern literature. Its susceptibility to oxidation, heat, and light necessitates specialized handling. Early preparation methods involved lyophilizing this compound solutions under nitrogen atmospheres to minimize degradation. However, these methods often yielded pink-tinged residues, indicative of partial oxidation and diminished therapeutic efficacy.
Modern Stabilization Techniques Using Reducing Sugars
Role of Reducing Sugars in Aqueous Solutions
The incorporation of reducing sugars, such as levulose (fructose), glucose, or lactose, has revolutionized this compound stabilization. These sugars act as auxiliary reducing agents, countering this compound’s oxidative degradation. A critical mass ratio of 5:1 to 25:1 (sugar-to-alloxan) is required to maintain stability, with levulose preferred for its superior solubility.
Mechanism of Action :
- Reducing sugars donate electrons to this compound, mitigating its oxidation by atmospheric oxygen.
- This protective effect is empirically validated by ammonia tests: stable solutions remain colorless upon ammonia addition, whereas degraded solutions turn pink.
Formulation Protocols for Injectable Solutions
For therapeutic applications, this compound is administered intramuscularly, necessitating sterile, stable injectables. The following protocol is widely adopted:
Solution Preparation :
- Dissolve 10 mg anhydrous this compound (or 12.27 mg monohydrate/15.07 mg tetrahydrate) per 1 mL water or physiological saline.
- Add 50 mg levulose per 1 mL solution (5:1 ratio for isotonicity).
Sterilization :
- Tyndallization : Heat ampules at 60–70°C to avoid thermal degradation.
- Membrane Filtration : Sterilize via bacteriological-grade filters at room temperature.
Packaging :
- Fill 5 mL ampules under sterile nitrogen atmospheres to preclude oxidative exposure.
Table 1: Sugar Selection and Stability Outcomes
Sugar | Ratio (Sugar:this compound) | Solubility (g/100 mL) | Thermal Stability |
---|---|---|---|
Levulose | 5:1–15:1 | 79.0 | High (70°C) |
Glucose | 10:1–25:1 | 47.0 | Moderate (60°C) |
Lactose | 15:1–25:1 | 18.0 | Low (50°C) |
Analytical Validation of this compound Preparations
Spectrophotometric and Voltammetric Assays
Recent advances enable indirect quantification via this compound derivatization. Reacting this compound with o-phenylenediamine (OPD) forms alloxazine, a stable adduct detectable at 610 nm spectrophotometrically or via cyclic voltammetry.
Procedure :
- Mix this compound solution with OPD in acetate buffer (pH 4.0).
- Incubate for 1 hour to form alloxazine.
- Quantify via peak current (DPV) or absorbance (UV-Vis).
Table 2: Analytical Performance Metrics
Method | Linear Range (μM) | LOD (μM) | LOQ (μM) |
---|---|---|---|
DPV | 10–600 | 2.1 | 6.9 |
UV-Vis | 50–500 | 15.3 | 50.1 |
Applications in Pharmaceutical Development
Therapeutic Formulations
Stable this compound solutions are pivotal in oncology (Groban therapy) and diabetes research. The levulose-based formulation remains the clinical benchmark due to its extended shelf-life (>24 months at 25°C).
Research Reagents
This compound’s role in inducing rodent diabetes necessitates batch consistency, achievable only through rigorous adherence to the above protocols.
Análisis De Reacciones Químicas
Redox Cycling with Dialuric Acid
Alloxan undergoes redox cycling with its reduced form, dialuric acid, generating reactive oxygen species (ROS) critical to its diabetogenic effects.
Mechanism :
-
Reduction : this compound is reduced to dialuric acid (HA⁻) via glutathione (GSH) or NADH, forming an intermediate this compound radical (AH- ) .
-
Autoxidation : Dialuric acid spontaneously reoxidizes back to this compound, producing superoxide radicals (O₂⁻) .
-
ROS Formation : Superoxide dismutates to hydrogen peroxide (H₂O₂), which generates hydroxyl radicals (- OH) via Fenton reactions, causing β-cell DNA damage .
Kinetic Data :
Reaction Step | Rate Constant (M⁻¹s⁻¹) | Conditions | Source |
---|---|---|---|
This compound + O₂⁻ → AH- | 3.4 × 10⁶ | pH 7.4, 25°C | |
Dialuric acid + SO₄- − → AH- | 8 × 10⁷ | pH 7.4, 25°C | |
AH- dimerization | 1.7 × 10⁸ | pH 7.4, 25°C |
Key Findings :
-
One this compound molecule oxidizes ~50 GSH molecules and produces ~25 H₂O₂ molecules per redox cycle .
-
At 250 μmol/L O₂, redox cycling frequency reaches ~33 cycles/hour, accelerating β-cell destruction .
Solvent-Dependent Hydration
This compound’s structure varies with solvent polarity, affecting its reactivity.
Hydration Pathways :
-
Protic Solvents (e.g., H₂O, Acetic Acid) :
-
Aprotic Solvents (e.g., DMSO) :
Thermodynamic Data :
Solvent | Major Species | Activation Energy (kcal/mol) | Source |
---|---|---|---|
Water | C5 gem-diol | ΔG‡ = 16 (reduced) | |
Acetic Acid | C5 gem-diol | ΔG‡ = 27 (reduced) | |
DMSO | Tetraketo + gem-diol | ΔG‡ = 43 (unassisted) |
Decomposition Pathways
This compound’s stability depends on environmental reductants and oxygen levels.
Decomposition Routes :
-
With GSH : Redox cycling dominates, delaying decomposition. Higher O₂ concentrations accelerate ROS production and GSH oxidation .
Oxygen Impact :
O₂ Concentration (μmol/L) | GSH Oxidation Rate | Dialuric Acid Accumulation | Source |
---|---|---|---|
0 (Argon) | None | High | |
25 | Moderate | Moderate | |
250 | Rapid | Low |
Metal Complexation Reactions
This compound forms stable complexes with transition metals, influencing its biological activity.
Synthesis :
-
Schiff-Base Complexes : this compound condenses with ligands like o-phenylenediamine (OPD) to form vanadium (VO²⁺) and zirconium (ZrO²⁺) complexes .
Thermal Stability (TGA Data) :
Complex | Decomposition Step (°C) | Mass Loss (%) | Residue | Source |
---|---|---|---|---|
VO(acac)L₁ | 301–369 | 73.5–39.4 | VO₂ | |
Zr(acac)₃L₁ | 290–325 | 83.0–48.8 | ZrO₂ |
Key Insight : Degradation of this compound moieties occurs above 325°C, leaving metal oxides as residues .
Electrochemical Detection
This compound’s redox activity enables sensitive voltammetric detection.
Method :
-
In Situ Reaction : this compound reacts with OPD to form electroactive alloxazine, detectable via cyclic voltammetry (CV) or differential pulse voltammetry (DPV) .
Performance Metrics :
Parameter | CV | DPV | Source |
---|---|---|---|
Linear Range (μM) | 0–600 | 0–600 | |
LOD (μM) | 1.86 | 1.95 | |
Sensitivity (μA/μM) | 0.032 | 0.029 |
Aplicaciones Científicas De Investigación
Key Applications
-
Diabetes Research
- Modeling Type 1 Diabetes : Alloxan is primarily used to create animal models that replicate type 1 diabetes. Studies have shown that this compound-induced diabetes results in significant biochemical changes, including elevated blood glucose levels and alterations in lipid profiles .
- Evaluating Antidiabetic Agents : Researchers utilize this compound-induced diabetic models to assess the efficacy of new antidiabetic drugs or natural compounds. For example, studies have demonstrated that various herbal extracts can ameliorate hyperglycemia and restore normal metabolic functions in this compound-treated rats .
-
Investigating Oxidative Stress
- This compound is instrumental in studying oxidative stress mechanisms associated with diabetes. It induces oxidative damage in tissues, allowing researchers to explore antioxidant therapies. For instance, treatment with antioxidants has been shown to reduce oxidative stress markers and improve pancreatic function in diabetic rats .
-
Immunological Studies
- Research has indicated that this compound-induced diabetes affects immune responses. Studies have demonstrated that diabetic mice show impaired immune function, which can be reversed by insulin treatment . This application is crucial for understanding the interplay between diabetes and immune system dysfunction.
- Pharmacological Investigations
Study on Butin's Effects
A study investigated butin's effects on this compound-induced diabetic rats. The results showed that butin significantly improved biochemical parameters such as blood glucose levels and antioxidant enzyme activities while reducing inflammatory markers . This indicates its potential as a therapeutic agent for managing diabetes-related complications.
Sida Cordata's Antidiabetic Properties
Another study focused on Sida cordata's efficacy against this compound-induced diabetes. The administration of this plant extract improved glucose tolerance and lipid profiles while enhancing antioxidant defenses in diabetic rats . This case underscores the importance of natural products in diabetes management.
Data Summary
Mecanismo De Acción
El aloxano ejerce sus efectos principalmente a través de la generación de especies reactivas de oxígeno (ROS). Al ingresar a las células beta pancreáticas a través del transportador de glucosa GLUT2, el aloxano experimenta un ciclo redox con su producto de reducción, el ácido dialúrico . Este ciclo genera radicales superóxido, peróxido de hidrógeno y radicales hidroxilo, que causan estrés oxidativo y daño a las células beta . Además, el aloxano inhibe la enzima glucoquinasa, lo que dificulta aún más la secreción de insulina .
Comparación Con Compuestos Similares
Alloxan vs. Ninhydrin
Structural and Functional Similarities :
- Both are oxidizing agents capable of degrading α-amino acids via the Strecker reaction in vitro .
- In buffered aqueous solutions, this compound and ninhydrin degrade alanine, with reaction rates influenced by aeration .
Key Differences :
Critical Insight : Despite shared oxidative properties, ninhydrin lacks diabetogenicity due to its inability to selectively accumulate in β-cells or inhibit glucokinase .
This compound vs. Streptozotocin (STZ)
Mechanistic Divergence :
Clinical Relevance : STZ is preferred for reproducible diabetes induction due to its stability, whereas this compound is pivotal for studying ROS-mediated β-cell pathology .
This compound vs. N-Alkylthis compound Derivatives
Example Compounds : Butylthis compound, methylthis compound.
Shared Features :
Critical Differences :
Implication : Lipophilic derivatives fail as diabetes inducers due to off-target renal toxicity, underscoring this compound’s unique balance of hydrophilicity and β-cell specificity .
Data Tables
Table 1: In Vitro Toxicity in Pancreatic Islets
Compound | β-Cell Viability (24 h) | Other Cell Viability |
---|---|---|
This compound (1 mM) | 10–20% | >80% |
Ninhydrin (1 mM) | 60–70% | 30–40% |
Butylthis compound (1 mM) | 15–25% | >75% |
Table 2: Blood Glucose Response in Rats
Compound (Dose) | Hyperglycemia Onset | Severity (Glucose mmol/L) |
---|---|---|
This compound (150 mg/kg) | 10–30 minutes | 25–30 |
STZ (55 mg/kg) | 2–4 hours | 20–25 |
Ninhydrin (150 mg/kg) | No effect | N/A |
Actividad Biológica
Alloxan is a compound extensively utilized in biomedical research, particularly for inducing diabetes in animal models. Its biological activity is primarily characterized by its selective cytotoxicity to pancreatic beta cells, leading to insulin-dependent diabetes mellitus (IDDM). This article explores the mechanisms, effects, and implications of this compound's biological activity based on diverse research findings.
This compound induces diabetes primarily through the following mechanisms:
- Cytotoxicity to Beta Cells : this compound selectively targets pancreatic beta cells, leading to their destruction. This results in decreased insulin production and subsequent hyperglycemia. The mechanism involves the generation of reactive oxygen species (ROS), which cause oxidative stress and cellular damage .
- Inhibition of Glucokinase : this compound has been shown to inhibit glucokinase activity in both pancreatic and hepatic tissues. This inhibition contributes to impaired glucose metabolism and exacerbates diabetic conditions .
Biological Effects
The biological effects of this compound are significant, particularly in experimental models:
- Induction of Diabetes : this compound is commonly used to induce diabetes in laboratory animals. For instance, a study demonstrated that a single intravenous injection of this compound (100 mg/kg) resulted in hyperglycemia and hypoinsulinism in mice .
- Oxidative Stress : this compound treatment leads to increased oxidative stress markers, including malondialdehyde (MDA), while decreasing antioxidant enzyme levels such as superoxide dismutase (SOD) and catalase (CAT) .
- Inflammatory Response : this compound-induced diabetes is associated with elevated levels of pro-inflammatory cytokines like TNF-α and IL-6, which contribute to further pancreatic damage .
Case Studies
Several studies have investigated the effects of this compound on various biological parameters:
Research Findings
Research has highlighted various aspects of this compound's biological activity:
- Diabetogenic Properties : this compound is widely recognized for its ability to induce diabetes through oxidative stress mechanisms. Its use as a diabetogenic agent has been validated across multiple studies, although some researchers argue about its consistency as a model for human diabetes .
- Potential Therapeutic Interventions : Recent studies have explored potential protective agents against this compound-induced damage. For instance, butin has demonstrated anti-inflammatory properties that mitigate the adverse effects of this compound on pancreatic function .
- Differences with Other Diabetogenic Agents : Comparisons between this compound and streptozotocin reveal differences in their mechanisms; while this compound primarily induces oxidative stress, streptozotocin acts through DNA alkylation .
Q & A
Basic Research Questions
Q. What is the mechanism by which Alloxan induces diabetes in experimental models?
this compound selectively destroys pancreatic β-cells through reactive oxygen species (ROS)-mediated cytotoxicity. Upon administration, this compound undergoes redox cycling with its reduced form, dialuric acid, generating superoxide anions, hydrogen peroxide, and hydroxyl radicals. These ROS damage β-cell DNA, disrupt calcium homeostasis, and induce apoptosis . For experimental validation, researchers often measure markers like glutathione depletion, ROS levels (e.g., via fluorescent probes), and histopathological changes in islet cells .
Q. How does this compound dosage vary across animal species, and what factors influence this variability?
Dosage depends on species susceptibility, administration route, and nutritional status. Example ranges:
Species | Dose (mg/kg) | Route |
---|---|---|
Rats | 40–200 | Intravenous (i.v.) |
Mice | 50–150 | Intraperitoneal (i.p.) |
Rabbits | 100–150 | i.v. |
Higher doses in resistant species (e.g., dogs) may require multiple administrations. Fasting animals for 12–24 hours pre-injection enhances efficacy due to reduced antioxidant defenses . |
Q. What criteria should guide the selection of animal models for this compound-induced diabetes studies?
- Species-specific β-cell sensitivity : Rats and mice are preferred for rapid β-cell necrosis, while rabbits exhibit delayed hyperglycemia.
- Disease severity : Single high doses (e.g., 150 mg/kg in rats) mimic acute type 1 diabetes, whereas subdiabetogenic doses combined with high-fat diets model type 2 diabetes .
- Ethical considerations : Use glucose supplementation to mitigate hypoglycemic shock mortality in rabbits .
Q. What are the advantages and limitations of different this compound administration routes?
- Intravenous (i.v.) : Ensures rapid bioavailability but requires surgical expertise.
- Intraperitoneal (i.p.) : Easier to perform but may cause peritoneal inflammation.
- Subcutaneous (s.c.) : Rarely used due to inconsistent absorption.
Validate success via fasting blood glucose (FBG) > 250 mg/dL sustained for 72 hours post-injection .
Advanced Research Questions
Q. How can researchers experimentally validate the role of ROS in this compound-induced β-cell damage?
- Inhibitor studies : Co-administer antioxidants (e.g., superoxide dismutase, catalase) or metal chelators (e.g., diethylenetriaminepentacetic acid) to block ROS generation. For example, 1000 U/mL superoxide dismutase reduces this compound’s inhibitory effect on glucose-stimulated insulin release .
- Biochemical assays : Quantify lipid peroxidation (MDA levels), DNA fragmentation (Comet assay), and caspase-3 activation .
Q. What interventions reduce mortality in this compound-induced diabetic models?
- Glucose supplementation : Administer 5% glucose intravenously (10 mL) and intraperitoneally (10 mL) every 4 hours during the hypoglycemic phase. This protocol reduced rabbit mortality from 60% to 20% in a 2024 study .
- Timing : Monitor blood glucose every 2 hours for the first 12 hours post-injection to detect hypoglycemia.
Q. How should researchers address contradictions in this compound’s triphasic blood glucose response?
The triphasic response includes:
Initial hyperglycemia (0–2 hours): Due to adrenergic stimulation.
Hypoglycemia (2–6 hours): From residual insulin release.
Persistent hyperglycemia (>24 hours): β-cell necrosis.
To resolve variability, standardize fasting protocols and exclude animals with FBG < 180 mg/dL after 72 hours .
Q. What methodologies ensure this compound-induced model validity for antidiabetic drug testing?
- Positive controls : Use glibenclamide (10 mg/kg) to confirm model responsiveness .
- Endpoint validation : Histologically confirm β-cell loss via hematoxylin-eosin staining or insulin immunohistochemistry .
- Long-term monitoring : Track FBG for 4 weeks to exclude spontaneous recovery .
Q. How can dose-response relationships be optimized for this compound in novel species?
Design a pilot study with escalating doses (e.g., 50–200 mg/kg) and monitor FBG at 24, 48, and 72 hours. Use survival curves and histopathology to identify the minimal diabetogenic dose. For example:
Dose (mg/kg) | % Diabetic (FBG > 250 mg/dL) | Mortality (%) |
---|---|---|
50 | 30 | 5 |
100 | 85 | 15 |
150 | 95 | 30 |
Adapted from . |
Q. Why does this compound selectively target β-cells, and how can this specificity be exploited?
this compound’s selectivity arises from:
Propiedades
IUPAC Name |
1,3-diazinane-2,4,5,6-tetrone | |
---|---|---|
Source | PubChem | |
URL | https://pubchem.ncbi.nlm.nih.gov | |
Description | Data deposited in or computed by PubChem | |
InChI |
InChI=1S/C4H2N2O4/c7-1-2(8)5-4(10)6-3(1)9/h(H2,5,6,8,9,10) | |
Source | PubChem | |
URL | https://pubchem.ncbi.nlm.nih.gov | |
Description | Data deposited in or computed by PubChem | |
InChI Key |
HIMXGTXNXJYFGB-UHFFFAOYSA-N | |
Source | PubChem | |
URL | https://pubchem.ncbi.nlm.nih.gov | |
Description | Data deposited in or computed by PubChem | |
Canonical SMILES |
C1(=O)C(=O)NC(=O)NC1=O | |
Source | PubChem | |
URL | https://pubchem.ncbi.nlm.nih.gov | |
Description | Data deposited in or computed by PubChem | |
Molecular Formula |
C4H2N2O4 | |
Source | PubChem | |
URL | https://pubchem.ncbi.nlm.nih.gov | |
Description | Data deposited in or computed by PubChem | |
DSSTOX Substance ID |
DTXSID5044946 | |
Record name | Alloxan | |
Source | EPA DSSTox | |
URL | https://comptox.epa.gov/dashboard/DTXSID5044946 | |
Description | DSSTox provides a high quality public chemistry resource for supporting improved predictive toxicology. | |
Molecular Weight |
142.07 g/mol | |
Source | PubChem | |
URL | https://pubchem.ncbi.nlm.nih.gov | |
Description | Data deposited in or computed by PubChem | |
Physical Description |
Solid; [Merck Index] Tetrahydrate: White solid; [Reidel-de Haen MSDS], Solid | |
Record name | Alloxan | |
Source | Haz-Map, Information on Hazardous Chemicals and Occupational Diseases | |
URL | https://haz-map.com/Agents/3563 | |
Description | Haz-Map® is an occupational health database designed for health and safety professionals and for consumers seeking information about the adverse effects of workplace exposures to chemical and biological agents. | |
Explanation | Copyright (c) 2022 Haz-Map(R). All rights reserved. Unless otherwise indicated, all materials from Haz-Map are copyrighted by Haz-Map(R). No part of these materials, either text or image may be used for any purpose other than for personal use. Therefore, reproduction, modification, storage in a retrieval system or retransmission, in any form or by any means, electronic, mechanical or otherwise, for reasons other than personal use, is strictly prohibited without prior written permission. | |
Record name | Alloxan | |
Source | Human Metabolome Database (HMDB) | |
URL | http://www.hmdb.ca/metabolites/HMDB0002818 | |
Description | The Human Metabolome Database (HMDB) is a freely available electronic database containing detailed information about small molecule metabolites found in the human body. | |
Explanation | HMDB is offered to the public as a freely available resource. Use and re-distribution of the data, in whole or in part, for commercial purposes requires explicit permission of the authors and explicit acknowledgment of the source material (HMDB) and the original publication (see the HMDB citing page). We ask that users who download significant portions of the database cite the HMDB paper in any resulting publications. | |
Boiling Point |
Sublimes | |
Record name | ALLOXAN | |
Source | Hazardous Substances Data Bank (HSDB) | |
URL | https://pubchem.ncbi.nlm.nih.gov/source/hsdb/7493 | |
Description | The Hazardous Substances Data Bank (HSDB) is a toxicology database that focuses on the toxicology of potentially hazardous chemicals. It provides information on human exposure, industrial hygiene, emergency handling procedures, environmental fate, regulatory requirements, nanomaterials, and related areas. The information in HSDB has been assessed by a Scientific Review Panel. | |
Solubility |
Sol in benzene, Soluble in acetone, alcohol, methanol, glacial acetic acid; slightly soluble in chloroform, petroleum ether, toluene, ethyl acetate and acetic anhydride. Insoluble in ether., Freely soluble in water. | |
Record name | ALLOXAN | |
Source | Hazardous Substances Data Bank (HSDB) | |
URL | https://pubchem.ncbi.nlm.nih.gov/source/hsdb/7493 | |
Description | The Hazardous Substances Data Bank (HSDB) is a toxicology database that focuses on the toxicology of potentially hazardous chemicals. It provides information on human exposure, industrial hygiene, emergency handling procedures, environmental fate, regulatory requirements, nanomaterials, and related areas. The information in HSDB has been assessed by a Scientific Review Panel. | |
Density |
1.07 | |
Record name | ALLOXAN | |
Source | Hazardous Substances Data Bank (HSDB) | |
URL | https://pubchem.ncbi.nlm.nih.gov/source/hsdb/7493 | |
Description | The Hazardous Substances Data Bank (HSDB) is a toxicology database that focuses on the toxicology of potentially hazardous chemicals. It provides information on human exposure, industrial hygiene, emergency handling procedures, environmental fate, regulatory requirements, nanomaterials, and related areas. The information in HSDB has been assessed by a Scientific Review Panel. | |
Color/Form |
Orthorhombic crystals from anhydrous acetone or glacial acetic acid or by sublimation in vacuo. Turns pink at 230 °C ... Hot aqueous solutions are yellow and become colorless on cooling | |
CAS No. |
50-71-5 | |
Record name | Alloxan | |
Source | CAS Common Chemistry | |
URL | https://commonchemistry.cas.org/detail?cas_rn=50-71-5 | |
Description | CAS Common Chemistry is an open community resource for accessing chemical information. Nearly 500,000 chemical substances from CAS REGISTRY cover areas of community interest, including common and frequently regulated chemicals, and those relevant to high school and undergraduate chemistry classes. This chemical information, curated by our expert scientists, is provided in alignment with our mission as a division of the American Chemical Society. | |
Explanation | The data from CAS Common Chemistry is provided under a CC-BY-NC 4.0 license, unless otherwise stated. | |
Record name | Alloxan | |
Source | ChemIDplus | |
URL | https://pubchem.ncbi.nlm.nih.gov/substance/?source=chemidplus&sourceid=0000050715 | |
Description | ChemIDplus is a free, web search system that provides access to the structure and nomenclature authority files used for the identification of chemical substances cited in National Library of Medicine (NLM) databases, including the TOXNET system. | |
Record name | Alloxan | |
Source | DTP/NCI | |
URL | https://dtp.cancer.gov/dtpstandard/servlet/dwindex?searchtype=NSC&outputformat=html&searchlist=7169 | |
Description | The NCI Development Therapeutics Program (DTP) provides services and resources to the academic and private-sector research communities worldwide to facilitate the discovery and development of new cancer therapeutic agents. | |
Explanation | Unless otherwise indicated, all text within NCI products is free of copyright and may be reused without our permission. Credit the National Cancer Institute as the source. | |
Record name | 2,4,5,6(1H,3H)-Pyrimidinetetrone | |
Source | EPA Chemicals under the TSCA | |
URL | https://www.epa.gov/chemicals-under-tsca | |
Description | EPA Chemicals under the Toxic Substances Control Act (TSCA) collection contains information on chemicals and their regulations under TSCA, including non-confidential content from the TSCA Chemical Substance Inventory and Chemical Data Reporting. | |
Record name | Alloxan | |
Source | EPA DSSTox | |
URL | https://comptox.epa.gov/dashboard/DTXSID5044946 | |
Description | DSSTox provides a high quality public chemistry resource for supporting improved predictive toxicology. | |
Record name | Alloxan | |
Source | European Chemicals Agency (ECHA) | |
URL | https://echa.europa.eu/substance-information/-/substanceinfo/100.000.057 | |
Description | The European Chemicals Agency (ECHA) is an agency of the European Union which is the driving force among regulatory authorities in implementing the EU's groundbreaking chemicals legislation for the benefit of human health and the environment as well as for innovation and competitiveness. | |
Explanation | Use of the information, documents and data from the ECHA website is subject to the terms and conditions of this Legal Notice, and subject to other binding limitations provided for under applicable law, the information, documents and data made available on the ECHA website may be reproduced, distributed and/or used, totally or in part, for non-commercial purposes provided that ECHA is acknowledged as the source: "Source: European Chemicals Agency, http://echa.europa.eu/". Such acknowledgement must be included in each copy of the material. ECHA permits and encourages organisations and individuals to create links to the ECHA website under the following cumulative conditions: Links can only be made to webpages that provide a link to the Legal Notice page. | |
Record name | ALLOXAN | |
Source | FDA Global Substance Registration System (GSRS) | |
URL | https://gsrs.ncats.nih.gov/ginas/app/beta/substances/6SW5YHA5NG | |
Description | The FDA Global Substance Registration System (GSRS) enables the efficient and accurate exchange of information on what substances are in regulated products. Instead of relying on names, which vary across regulatory domains, countries, and regions, the GSRS knowledge base makes it possible for substances to be defined by standardized, scientific descriptions. | |
Explanation | Unless otherwise noted, the contents of the FDA website (www.fda.gov), both text and graphics, are not copyrighted. They are in the public domain and may be republished, reprinted and otherwise used freely by anyone without the need to obtain permission from FDA. Credit to the U.S. Food and Drug Administration as the source is appreciated but not required. | |
Record name | ALLOXAN | |
Source | Hazardous Substances Data Bank (HSDB) | |
URL | https://pubchem.ncbi.nlm.nih.gov/source/hsdb/7493 | |
Description | The Hazardous Substances Data Bank (HSDB) is a toxicology database that focuses on the toxicology of potentially hazardous chemicals. It provides information on human exposure, industrial hygiene, emergency handling procedures, environmental fate, regulatory requirements, nanomaterials, and related areas. The information in HSDB has been assessed by a Scientific Review Panel. | |
Record name | Alloxan | |
Source | Human Metabolome Database (HMDB) | |
URL | http://www.hmdb.ca/metabolites/HMDB0002818 | |
Description | The Human Metabolome Database (HMDB) is a freely available electronic database containing detailed information about small molecule metabolites found in the human body. | |
Explanation | HMDB is offered to the public as a freely available resource. Use and re-distribution of the data, in whole or in part, for commercial purposes requires explicit permission of the authors and explicit acknowledgment of the source material (HMDB) and the original publication (see the HMDB citing page). We ask that users who download significant portions of the database cite the HMDB paper in any resulting publications. | |
Melting Point |
256 °C (decomposes), 256 °C | |
Record name | ALLOXAN | |
Source | Hazardous Substances Data Bank (HSDB) | |
URL | https://pubchem.ncbi.nlm.nih.gov/source/hsdb/7493 | |
Description | The Hazardous Substances Data Bank (HSDB) is a toxicology database that focuses on the toxicology of potentially hazardous chemicals. It provides information on human exposure, industrial hygiene, emergency handling procedures, environmental fate, regulatory requirements, nanomaterials, and related areas. The information in HSDB has been assessed by a Scientific Review Panel. | |
Record name | Alloxan | |
Source | Human Metabolome Database (HMDB) | |
URL | http://www.hmdb.ca/metabolites/HMDB0002818 | |
Description | The Human Metabolome Database (HMDB) is a freely available electronic database containing detailed information about small molecule metabolites found in the human body. | |
Explanation | HMDB is offered to the public as a freely available resource. Use and re-distribution of the data, in whole or in part, for commercial purposes requires explicit permission of the authors and explicit acknowledgment of the source material (HMDB) and the original publication (see the HMDB citing page). We ask that users who download significant portions of the database cite the HMDB paper in any resulting publications. | |
Retrosynthesis Analysis
AI-Powered Synthesis Planning: Our tool employs the Template_relevance Pistachio, Template_relevance Bkms_metabolic, Template_relevance Pistachio_ringbreaker, Template_relevance Reaxys, Template_relevance Reaxys_biocatalysis model, leveraging a vast database of chemical reactions to predict feasible synthetic routes.
One-Step Synthesis Focus: Specifically designed for one-step synthesis, it provides concise and direct routes for your target compounds, streamlining the synthesis process.
Accurate Predictions: Utilizing the extensive PISTACHIO, BKMS_METABOLIC, PISTACHIO_RINGBREAKER, REAXYS, REAXYS_BIOCATALYSIS database, our tool offers high-accuracy predictions, reflecting the latest in chemical research and data.
Strategy Settings
Precursor scoring | Relevance Heuristic |
---|---|
Min. plausibility | 0.01 |
Model | Template_relevance |
Template Set | Pistachio/Bkms_metabolic/Pistachio_ringbreaker/Reaxys/Reaxys_biocatalysis |
Top-N result to add to graph | 6 |
Feasible Synthetic Routes
Descargo de responsabilidad e información sobre productos de investigación in vitro
Tenga en cuenta que todos los artículos e información de productos presentados en BenchChem están destinados únicamente con fines informativos. Los productos disponibles para la compra en BenchChem están diseñados específicamente para estudios in vitro, que se realizan fuera de organismos vivos. Los estudios in vitro, derivados del término latino "in vidrio", involucran experimentos realizados en entornos de laboratorio controlados utilizando células o tejidos. Es importante tener en cuenta que estos productos no se clasifican como medicamentos y no han recibido la aprobación de la FDA para la prevención, tratamiento o cura de ninguna condición médica, dolencia o enfermedad. Debemos enfatizar que cualquier forma de introducción corporal de estos productos en humanos o animales está estrictamente prohibida por ley. Es esencial adherirse a estas pautas para garantizar el cumplimiento de los estándares legales y éticos en la investigación y experimentación.