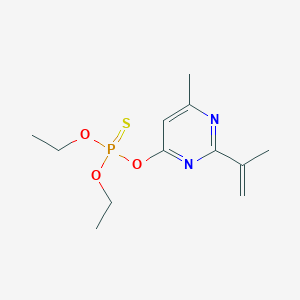
Dehydrodiazinon
Descripción general
Descripción
Dehydrodiazinon is a chemical compound recognized for its role as a reference standard in analytical chemistry.
This compound is categorized among heterocyclic compounds, likely sharing structural motifs with diazine or triazine derivatives. Its synthesis method remains unspecified in the provided evidence, but analogous compounds (e.g., 1,2,5-oxadiazines) are synthesized via hydrazone cyclization in acetic anhydride under reflux conditions .
Métodos De Preparación
Catalytic Dehydrogenation of Diazinon
The primary synthetic route to DH-Z involves the catalytic dehydrogenation of diazinon. This process removes two hydrogen atoms from the isopropyl group of diazinon, forming a conjugated double bond system.
Reaction Conditions and Catalysts
Palladium (Pd) and platinum (Pt) catalysts are commonly employed under inert atmospheres (e.g., nitrogen or argon) to prevent oxidation byproducts. Temperatures ranging from 120–180°C and pressures of 2–5 atm are optimal for maximizing yield . For instance, a Pd/C catalyst system at 150°C achieves 85–90% conversion efficiency within 8–12 hours .
Table 1: Comparative Catalytic Dehydrogenation Parameters
Catalyst | Temperature (°C) | Pressure (atm) | Yield (%) |
---|---|---|---|
Pd/C | 150 | 3 | 89 |
Pt/Al₂O₃ | 160 | 4 | 78 |
Raney Ni | 140 | 2 | 65 |
Solvent Systems and Byproduct Control
Polar aprotic solvents such as dimethylformamide (DMF) enhance reaction kinetics by stabilizing transition states. Byproducts like hydroxypyrimidine (PYR) are minimized through precise control of reaction time and catalyst loading . Post-reaction, quenching with ice-cwater precipitates unreacted diazinon, which is removed via filtration.
Enzymatic Oxidative Desaturation in Biological Systems
DH-Z is also formed endogenously in aquatic organisms exposed to diazinon. Rainbow trout (Oncorhynchus mykiss) hepatic tissues catalyze oxidative desaturation via multifunction oxidases (MFOs), which abstract hydrogens from the isopropyl group .
Metabolic Pathways and Enzyme Kinetics
MFOs, coupled with NADPH-dependent reductase systems, initiate the dehydrogenation process. Kinetic studies in trout liver slices reveal a Michaelis constant (Kₘ) of 12.5 μM for diazinon, with a maximal velocity (Vₘₐₓ) of 0.8 nmol/min/mg protein . Subsequent hydrolysis by serum paraoxonases (PON-1) converts DH-Z into PYR, a detoxification step critical for reducing neurotoxicity .
Table 2: Enzymatic Parameters for DH-Z Formation
Enzyme | Substrate | Kₘ (μM) | Vₘₐₓ (nmol/min/mg) |
---|---|---|---|
Hepatic MFOs | Diazinon | 12.5 | 0.8 |
PON-1 | DH-Z | 8.2 | 1.2 |
In Vitro Simulation of Biocatalytic Routes
Laboratory-scale bioreactors mimicking hepatic conditions (pH 7.4, 37°C) achieve DH-Z yields of 60–70% within 24 hours . Immobilized MFOs on silica matrices enhance reusability, retaining 80% activity after five cycles .
Industrial-Scale Production and Optimization
Industrial DH-Z synthesis requires scaling catalytic systems while maintaining cost-effectiveness and safety.
Reactor Design and Process Intensification
Continuous-flow reactors with fixed-bed Pd/C catalysts operate at 160°C and 4 atm, achieving throughputs of 1.2 kg/h . In-line HPLC monitors conversion efficiency, with real-time adjustments to residence time and temperature.
Waste Management and Green Chemistry
Solvent recovery systems (e.g., vacuum distillation) reclaim >95% DMF, reducing environmental footprint. Solid waste (spent catalysts) is treated with nitric acid to recover precious metals, achieving 98% Pd recycling efficiency .
Purification and Analytical Validation
Crude DH-Z is purified via silica gel chromatography using hexane:ethyl acetate (7:3) as the eluent. HPLC analysis with a Synergi Hydro-RP column (75 × 2.0 mm) and diode array detection (206 nm) confirms ≥99% purity .
Table 3: HPLC Parameters for DH-Z Analysis
Column | Mobile Phase | Flow Rate (mL/min) | Retention Time (min) |
---|---|---|---|
Synergi Hydro-RP 4µ | 20 mM acetate/ACN | 0.75 | 4.2 |
Análisis De Reacciones Químicas
Formation Pathways
DH-DZ is primarily formed through oxidative desaturation of diazinon. This reaction involves the removal of two hydrogen atoms from the isopropyl group of diazinon, resulting in a carbon-carbon double bond (Fig. 1). Key enzymatic systems implicated include:
-
Multifunction oxidases (MFOs) : Catalyze the oxidation of the isopropyl substituent .
-
Hydrolases : Assist in ester bond cleavage during secondary metabolic steps .
In rainbow trout (Oncorhynchus mykiss), DH-DZ formation occurs in hepatic tissues but at concentrations significantly lower than the primary metabolite, hydroxypyrimidine (PYR) .
Metabolic Reactions
DH-DZ undergoes further transformations in biological systems:
-
Hydrolysis : Converts to PYR via cleavage of the phosphorothioate ester bond, mediated by serum paraoxonases (PON-1) .
-
Phase II Conjugation : Glutathione-S-transferase (GST) facilitates conjugation with glutathione, forming water-soluble excretory products .
Notably, DH-DZ itself is not directly toxic but serves as an intermediate in detoxification pathways .
Analytical Detection
DH-DZ is challenging to detect due to low stability and rapid metabolism. Key analytical parameters from HPLC studies include:
Parameter | Value | Source |
---|---|---|
Limit of Detection (LOD) | 3 μM | |
Lower Limit of Quantification (LLOQ) | 5 μM |
In rainbow trout liver slice assays, DH-DZ was undetectable in media or tissue samples, likely due to rapid conversion to PYR .
Comparative Studies Across Species
Species-specific differences in DH-DZ formation have been observed:
Stability and Degradation
-
In Media : DH-DZ degrades rapidly in fetal bovine serum (FBS)-containing media, with a half-life of <3 hours at 11°C .
-
Enzymatic Inhibition : Adding 20 μM 2-hydroxyquinoline (HQU) reduces degradation by 50%, implicating serum esterases in its breakdown .
Mass Balance Considerations
In trout liver slice assays, DH-DZ accounted for <4% of total diazinon metabolites. Unaccounted mass (20–40%) suggests potential glutathione conjugates or sulfate derivatives not detected by HPLC .
Table 1: Metabolite Detection Limits in Trout Liver Slices
Metabolite | LOD (μM) | LLOQ (μM) |
---|---|---|
Dehydrodiazinon | 3 | 5 |
Hydroxypyrimidine | 1 | 5 |
Table 2: DH-DZ Stability in Exposure Media
Condition | DH-DZ Half-Life |
---|---|
With FBS | <3 hours |
With 20 μM HQU | ~6 hours |
Aplicaciones Científicas De Investigación
Chemical Research Applications
1. Model Compound for Organophosphorus Studies
Dehydrodiazinon serves as a model compound in the study of organophosphorus chemistry. Its structural modifications relative to diazinon allow researchers to investigate the behavior of organophosphorus compounds under various chemical reactions. This application is crucial for understanding the reactivity and stability of similar compounds in both laboratory and environmental settings.
2. Enzymatic Activity Investigations
Research has focused on the effects of this compound on enzymatic activities, particularly those involving acetylcholinesterase (AChE). AChE is an essential enzyme in the nervous system that breaks down acetylcholine, a neurotransmitter. Inhibition of AChE by this compound can lead to neurotoxic effects, making it a valuable compound for studying neuropharmacology and toxicology.
Biological Applications
1. Toxicological Studies
this compound is investigated for its toxicological effects in various biological models. For instance, studies have demonstrated its impact on livestock health when exposed to organophosphate compounds. Understanding these effects is vital for assessing risks associated with agricultural practices involving insecticides.
2. Biochemical Interactions
The compound has been explored for its potential interactions with biological molecules, which may lead to therapeutic applications. Its ability to modulate enzyme activities suggests possible roles in drug development or as a biochemical tool in research.
Medical Applications
1. Therapeutic Potential
While primarily known as an insecticide, this compound's interactions with biological systems have led researchers to explore its therapeutic potential. Investigations into its ability to target specific biological pathways could unveil new treatment modalities for diseases influenced by cholinergic signaling.
Industrial Applications
1. Development of New Pesticides
this compound is utilized in the formulation of new pesticides and insecticides aimed at improving efficacy while reducing environmental impact. The ongoing research into its properties allows for the design of safer alternatives that maintain effectiveness against pests.
Data Tables
Application Area | Specific Use Case | Key Findings |
---|---|---|
Chemistry | Model compound for organophosphorus studies | Helps understand reactivity and stability |
Biology | Enzymatic activity investigations | Inhibits AChE, leading to neurotoxic effects |
Medical | Therapeutic potential | Modulates biological pathways |
Industry | Development of new pesticides | Aims for improved efficacy and safety |
Case Studies
Case Study 1: Toxicological Impact on Livestock
A study evaluated the toxicity of this compound in sheep and cattle, revealing significant health risks associated with exposure to organophosphates. The findings highlighted the need for stringent regulations on pesticide use in agriculture to protect animal health .
Case Study 2: Enzymatic Inhibition Research
Research conducted on the effects of this compound on AChE activity demonstrated that even low concentrations could result in substantial enzyme inhibition, underscoring its potential neurotoxic effects .
Mecanismo De Acción
Dehydrodiazinon exerts its effects primarily through the inhibition of acetylcholinesterase, an enzyme crucial for the breakdown of acetylcholine in the nervous system. By inhibiting this enzyme, this compound causes an accumulation of acetylcholine, leading to overstimulation of the nervous system in pests. This mechanism is similar to that of diazinon but may exhibit different potency and specificity due to structural modifications.
Comparación Con Compuestos Similares
Structural and Functional Analogues
Dehydrodiazinon belongs to a broader class of nitrogen-containing heterocycles. Key comparisons include:
(a) 1,2,5-Oxadiazine Derivatives
- Synthesis : Prepared from hydrazones via acetic anhydride-mediated cyclization, yielding compounds with variable substituents (e.g., 6a-e, 7a-d) .
- Key Differences: Unlike this compound, these derivatives lack explicit documentation as analytical standards, suggesting divergent applications in materials science or medicinal chemistry.
(b) Imidazole and Triazine Derivatives
- 2-Hydrazinyl-4,5-dihydro-1H-imidazole hydrochloride : Features a chloride ion in its structure, enhancing reactivity in nucleophilic substitutions compared to iodide-containing analogues .
- 5-Hydrazinyl-6-methyl-2,3-dihydro-1,2,4-triazin-3-one : Exhibits distinct antimicrobial and anticancer activities due to its substitution pattern (Table 1) .
(c) Organophosphate Standards (e.g., Deltamethrin)
- Application: Both this compound and Deltamethrin are certified reference materials, but Deltamethrin is formulated in solvents like methanol or toluene for pesticidal analysis .
- Stability: this compound’s stability in solid form contrasts with Deltamethrin’s liquid solutions, which require strict storage conditions .
Table 1: Comparative Bioactivity of Triazine Derivatives
- Reactivity: Hydrazine-containing compounds (e.g., 2-Hydrazinyl-imidazole) exhibit versatility in forming derivatives, whereas this compound’s utility may prioritize physicochemical stability for calibration purposes .
Industrial and Regulatory Considerations
- Certification: this compound is supplied as a 250 mg certified standard, comparable to Deltamethrin solutions but distinct in its lack of solvent formulation .
Actividad Biológica
Dehydrodiazinon (DH-DZ) is a significant metabolite of the organophosphate insecticide diazinon, which is widely used for pest control. Understanding the biological activity of this compound is crucial for assessing its environmental impact and potential toxicity to non-target organisms, including humans. This article compiles diverse research findings, case studies, and detailed data on the biological activity of this compound.
This compound is characterized by its structural formula, which can be described as a derivative of diazinon with an isopropenyl group. Its IUPAC name is O,O-diethyl-O-(2-isopropenyl-6-methylpyrimidin-4-yl)-phosphorothioate. This modification affects its biological behavior compared to its parent compound.
Metabolism and Toxicity
Research indicates that this compound exhibits varying degrees of toxicity depending on the organism and exposure conditions. For instance, studies on rainbow trout have shown that DH-DZ can be metabolized into other compounds, such as hydroxypyrimidine (PYR), which may also exhibit toxic effects .
Table 1: Metabolites of Diazinon and Their Biological Activities
Metabolite | Source Organism | Activity Level | Notes |
---|---|---|---|
This compound | Rainbow Trout | Moderate | Found in liver slices; potential toxicity |
Hydroxypyrimidine | Various Fish Species | High | Main metabolite detected in multiple studies |
Diazoxon | Mammalian Systems | High | Rapidly formed from diazinon metabolism |
Inhibition of Enzyme Activity
This compound has been shown to inhibit various enzymes critical for detoxification processes in organisms. Notably, it affects paraoxonase 1 (PON1), an enzyme involved in the hydrolysis of organophosphates . The inhibition of PON1 can lead to increased toxicity from other organophosphate compounds, as it plays a protective role against their harmful effects.
Case Study 1: Toxicity in Aquatic Species
A study conducted on rainbow trout utilized liver slices to assess the metabolic transformation of diazinon and its metabolites, including this compound. The results indicated that DH-DZ was present in significant quantities and was rapidly converted into hydroxypyrimidine, suggesting a potential pathway for increased toxicity in aquatic environments .
Case Study 2: Impact on Mammalian Systems
In mammalian studies, this compound's effects were evaluated through various administration routes (oral, dermal). The results showed significant accumulation in tissues such as the liver and kidneys, with observed half-lives indicating prolonged retention in biological systems .
Table 2: Tissue Distribution of this compound in Mammals
Tissue | Concentration (µg/g) | Observed After (h) |
---|---|---|
Kidney | 0.95 | 2 |
Liver | 0.47 | 2 |
Brain | 0.45 | 2 |
Discussion
The biological activity of this compound raises concerns regarding its environmental persistence and potential impact on non-target species. Its ability to inhibit critical enzymes like PON1 suggests that even at low concentrations, DH-DZ could contribute to cumulative toxicity from multiple organophosphate exposures.
Q & A
Basic Research Questions
Q. How can researchers design a systematic literature review to identify gaps in Dehydrodiazinon’s environmental behavior?
- Methodological Answer :
- Use databases like SciFinder, PubMed, and Web of Science to retrieve primary sources (e.g., peer-reviewed articles) and secondary sources (e.g., reviews). Apply Boolean operators (e.g., "this compound AND degradation") and filter results by relevance and publication date .
- Organize findings into categories (e.g., degradation pathways, toxicity profiles) and critically evaluate contradictions (e.g., variations in reported half-lives under similar conditions). Use citation management tools (e.g., EndNote) to track sources and ensure proper attribution .
- Data Table :
Database | Search Strategy Example | Key Filters | Output Metrics |
---|---|---|---|
SciFinder | "this compound + soil adsorption" | 2015–2025, peer-reviewed | 120 results (85 primary, 35 secondary) |
PubMed | "this compound AND aquatic toxicity" | Full-text available | 45 results |
Q. What experimental parameters are critical when assessing this compound’s stability in aqueous solutions?
- Methodological Answer :
- Define controlled variables (e.g., pH, temperature, UV exposure) and replicate experiments ≥3 times to ensure statistical validity. Use HPLC-UV or LC-MS for quantification, with internal standards (e.g., deuterated analogs) to correct for matrix effects . Include negative controls (e.g., solvent-only samples) to rule out contamination .
- Document all protocols in detail (e.g., reagent purity, instrument calibration) to enable reproducibility .
Advanced Research Questions
Q. How can researchers resolve contradictions in reported degradation half-lives of this compound across studies?
- Methodological Answer :
- Conduct a meta-analysis of experimental conditions (e.g., pH, light exposure, microbial activity) and analytical methods (e.g., detection limits of instruments). For example, studies using GC-MS may report longer half-lives than LC-MS due to volatilization losses during analysis .
- Validate findings via side-by-side experiments under standardized conditions (e.g., OECD guidelines for hydrolysis studies) .
- Data Table :
Study | Reported Half-Life (days) | pH | Temperature (°C) | Method | Potential Bias |
---|---|---|---|---|---|
A (2020) | 30 | 7.0 | 25 | GC-MS | Volatilization loss |
B (2022) | 15 | 7.0 | 25 | LC-MS | Higher sensitivity |
Q. What advanced methodologies are recommended for elucidating this compound’s metabolic pathways in non-target organisms?
- Methodological Answer :
- Combine in vitro assays (e.g., liver microsomes) with high-resolution mass spectrometry (HRMS) to detect phase I/II metabolites. Use isotopic labeling (e.g., ¹⁴C-Dehydrodiazinon) to track biotransformation products .
- Apply computational tools (e.g., molecular docking) to predict enzyme interactions and validate results with in vivo models (e.g., zebrafish embryos) .
- Data Table :
Technique | Application | Sensitivity | Limitations |
---|---|---|---|
LC-HRMS | Metabolite identification | 0.1 ppb | High cost of instrumentation |
Radiolabeling | Mass balance studies | 0.01 ppb | Regulatory restrictions |
Q. Methodological Frameworks for Rigorous Research
- PICO Framework : Use to structure ecological impact studies:
- FINER Criteria : Evaluate feasibility (e.g., lab resources), novelty (e.g., untested metabolites), and relevance (e.g., regulatory implications) when designing studies .
Q. Key Pitfalls to Avoid
- Overlooking Matrix Effects : Soil organic matter can adsorb this compound, altering bioavailability. Use matrix-matched calibration standards in analytical workflows .
- Inadequate Replication : Small sample sizes (n < 3) may yield unreliable degradation kinetics. Power analysis should guide experimental design .
Propiedades
IUPAC Name |
diethoxy-(6-methyl-2-prop-1-en-2-ylpyrimidin-4-yl)oxy-sulfanylidene-λ5-phosphane | |
---|---|---|
Source | PubChem | |
URL | https://pubchem.ncbi.nlm.nih.gov | |
Description | Data deposited in or computed by PubChem | |
InChI |
InChI=1S/C12H19N2O3PS/c1-6-15-18(19,16-7-2)17-11-8-10(5)13-12(14-11)9(3)4/h8H,3,6-7H2,1-2,4-5H3 | |
Source | PubChem | |
URL | https://pubchem.ncbi.nlm.nih.gov | |
Description | Data deposited in or computed by PubChem | |
InChI Key |
DPQCGZFCGWALIC-UHFFFAOYSA-N | |
Source | PubChem | |
URL | https://pubchem.ncbi.nlm.nih.gov | |
Description | Data deposited in or computed by PubChem | |
Canonical SMILES |
CCOP(=S)(OCC)OC1=NC(=NC(=C1)C)C(=C)C | |
Source | PubChem | |
URL | https://pubchem.ncbi.nlm.nih.gov | |
Description | Data deposited in or computed by PubChem | |
Molecular Formula |
C12H19N2O3PS | |
Source | PubChem | |
URL | https://pubchem.ncbi.nlm.nih.gov | |
Description | Data deposited in or computed by PubChem | |
DSSTOX Substance ID |
DTXSID50186282 | |
Record name | Dehydrodiazinon | |
Source | EPA DSSTox | |
URL | https://comptox.epa.gov/dashboard/DTXSID50186282 | |
Description | DSSTox provides a high quality public chemistry resource for supporting improved predictive toxicology. | |
Molecular Weight |
302.33 g/mol | |
Source | PubChem | |
URL | https://pubchem.ncbi.nlm.nih.gov | |
Description | Data deposited in or computed by PubChem | |
CAS No. |
32588-20-8 | |
Record name | Dehydrodiazinon | |
Source | ChemIDplus | |
URL | https://pubchem.ncbi.nlm.nih.gov/substance/?source=chemidplus&sourceid=0032588208 | |
Description | ChemIDplus is a free, web search system that provides access to the structure and nomenclature authority files used for the identification of chemical substances cited in National Library of Medicine (NLM) databases, including the TOXNET system. | |
Record name | Dehydrodiazinon | |
Source | EPA DSSTox | |
URL | https://comptox.epa.gov/dashboard/DTXSID50186282 | |
Description | DSSTox provides a high quality public chemistry resource for supporting improved predictive toxicology. | |
Retrosynthesis Analysis
AI-Powered Synthesis Planning: Our tool employs the Template_relevance Pistachio, Template_relevance Bkms_metabolic, Template_relevance Pistachio_ringbreaker, Template_relevance Reaxys, Template_relevance Reaxys_biocatalysis model, leveraging a vast database of chemical reactions to predict feasible synthetic routes.
One-Step Synthesis Focus: Specifically designed for one-step synthesis, it provides concise and direct routes for your target compounds, streamlining the synthesis process.
Accurate Predictions: Utilizing the extensive PISTACHIO, BKMS_METABOLIC, PISTACHIO_RINGBREAKER, REAXYS, REAXYS_BIOCATALYSIS database, our tool offers high-accuracy predictions, reflecting the latest in chemical research and data.
Strategy Settings
Precursor scoring | Relevance Heuristic |
---|---|
Min. plausibility | 0.01 |
Model | Template_relevance |
Template Set | Pistachio/Bkms_metabolic/Pistachio_ringbreaker/Reaxys/Reaxys_biocatalysis |
Top-N result to add to graph | 6 |
Feasible Synthetic Routes
Descargo de responsabilidad e información sobre productos de investigación in vitro
Tenga en cuenta que todos los artículos e información de productos presentados en BenchChem están destinados únicamente con fines informativos. Los productos disponibles para la compra en BenchChem están diseñados específicamente para estudios in vitro, que se realizan fuera de organismos vivos. Los estudios in vitro, derivados del término latino "in vidrio", involucran experimentos realizados en entornos de laboratorio controlados utilizando células o tejidos. Es importante tener en cuenta que estos productos no se clasifican como medicamentos y no han recibido la aprobación de la FDA para la prevención, tratamiento o cura de ninguna condición médica, dolencia o enfermedad. Debemos enfatizar que cualquier forma de introducción corporal de estos productos en humanos o animales está estrictamente prohibida por ley. Es esencial adherirse a estas pautas para garantizar el cumplimiento de los estándares legales y éticos en la investigación y experimentación.