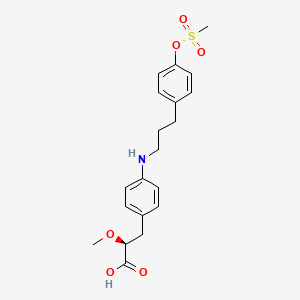
PPAR agonist 1
Descripción general
Descripción
Peroxisome proliferator-activated receptor agonist 1 is a compound that acts upon the peroxisome proliferator-activated receptor. These receptors are nuclear hormone receptors that play a crucial role in regulating gene expression related to energy metabolism, cellular development, and differentiation. Peroxisome proliferator-activated receptor agonist 1 is used in the treatment of metabolic syndrome, primarily for lowering triglycerides and blood sugar levels .
Métodos De Preparación
The synthesis of peroxisome proliferator-activated receptor agonist 1 involves several steps. The synthetic routes typically include the preparation of intermediate compounds, followed by their conversion into the final product under specific reaction conditions. Industrial production methods often involve large-scale synthesis using optimized reaction conditions to ensure high yield and purity .
Análisis De Reacciones Químicas
Peroxisome proliferator-activated receptor agonist 1 undergoes various chemical reactions, including oxidation, reduction, and substitution reactions. Common reagents used in these reactions include oxidizing agents, reducing agents, and nucleophiles. The major products formed from these reactions depend on the specific conditions and reagents used .
Aplicaciones Científicas De Investigación
Applications in Metabolic Disorders
PPAR agonists are extensively studied for their role in managing metabolic disorders, particularly:
Diabetes Management
PPARγ agonists, such as thiazolidinediones (TZDs), have been shown to enhance insulin sensitivity and improve glycemic control in type 2 diabetes patients. A meta-analysis indicated that these agents significantly reduce fasting blood glucose levels and HbA1c, demonstrating their efficacy in diabetes management .
Obesity Treatment
Research indicates that PPARγ activation promotes adipogenesis and enhances lipid storage, which can be beneficial in obesity management by improving metabolic parameters. Studies have shown that natural product agonists can improve metabolic outcomes in diabetic animal models with fewer side effects compared to synthetic TZDs .
Cardiovascular Health
PPARγ agonists have been linked to cardiovascular benefits through their anti-inflammatory properties and effects on lipid metabolism. For instance, pioglitazone has been shown to reduce palmitate-induced endoplasmic reticulum (ER) stress and apoptosis in macrophages, potentially lowering cardiovascular risk .
Cancer Research
Recent studies suggest that PPARγ agonists may possess antitumor effects across various cancer types. Evidence from in vitro studies indicates that both synthetic and natural PPARγ agonists can inhibit tumor growth by modulating cell cycle regulation and apoptosis pathways .
Neurological Applications
Research has highlighted the role of PPARγ in neuroinflammation and neuroprotection. Agonists like rosiglitazone have been shown to reduce microglial activation and pro-inflammatory cytokine production in models of neuroinflammatory diseases . This suggests potential therapeutic avenues for conditions such as multiple sclerosis or Alzheimer's disease.
Clinical Trials
A series of randomized controlled trials (RCTs) have demonstrated the safety and efficacy of PPAR agonists in patients with primary biliary cholangitis (PBC). These trials reported significant improvements in biochemical markers such as alkaline phosphatase (ALP) levels among patients treated with PPAR agonists compared to placebo .
Animal Models
In animal studies, PPARγ agonists have been shown to mitigate obesity-related complications by improving insulin sensitivity and reducing systemic inflammation. For example, administration of pioglitazone in obese mice resulted in enhanced beta-cell function and prevention of diabetes onset .
Data Table: Summary of Key Findings on PPAR Agonist Applications
Mecanismo De Acción
Peroxisome proliferator-activated receptor agonist 1 exerts its effects by binding to the peroxisome proliferator-activated receptor, which then forms a heterodimer with the retinoid X receptorThe molecular targets and pathways involved include genes related to lipid metabolism, glucose homeostasis, and inflammation .
Comparación Con Compuestos Similares
Peroxisome proliferator-activated receptor agonist 1 is unique in its high selectivity and potency compared to other similar compounds. Similar compounds include other peroxisome proliferator-activated receptor agonists such as fibrates and thiazolidinediones. These compounds also target peroxisome proliferator-activated receptors but may have different safety profiles and clinical outcomes .
Actividad Biológica
Peroxisome proliferator-activated receptors (PPARs) are a group of nuclear receptor proteins that function as transcription factors regulating the expression of genes involved in glucose and lipid metabolism, inflammation, and cellular differentiation. Among the PPAR family, PPARγ is particularly significant due to its role in insulin sensitivity and adipogenesis. This article focuses on the biological activity of PPAR agonist 1, exploring its mechanisms, effects, and potential therapeutic applications.
PPAR agonists, including this compound, primarily exert their effects by binding to PPAR receptors, leading to the activation of transcriptional programs that modulate metabolic processes. The binding of an agonist to PPAR induces a conformational change that allows the receptor to recruit coactivators and initiate gene transcription. This process is crucial for regulating lipid metabolism and glucose homeostasis.
Key Mechanisms Include:
- Alteration of Fatty Acid Metabolism: PPAR agonists enhance the uptake and utilization of nonesterified fatty acids (NEFA) in tissues such as adipose tissue and muscle, thereby improving insulin sensitivity .
- Regulation of Inflammatory Responses: PPARγ activation has been shown to have anti-inflammatory effects, which are beneficial in conditions like obesity and type 2 diabetes .
- Impact on Gene Expression: Agonists can differentially modulate the expression of genes involved in lipid metabolism, inflammation, and cell proliferation .
Clinical Efficacy
Research indicates that this compound demonstrates significant clinical efficacy in managing conditions such as type 2 diabetes (T2D) and metabolic syndrome. A systematic review highlighted the benefits of adding PPAR agonists to metformin therapy, showing improvements in glycemic control without significant adverse effects .
Table 1: Clinical Outcomes with PPAR Agonist Treatment
Parameter | Metformin Alone | Metformin + PPAR Agonist | Mean Difference (MD) |
---|---|---|---|
HbA1c (%) | Baseline | Reduced | -0.53% (95% CI: -0.67, -0.38) |
Fasting Insulin (pmol/L) | Baseline | Reduced | -19.83 (95% CI: -29.54, -10.13) |
HOMA-IR | Baseline | Reduced | -1.26 (95% CI: -2.16, -0.37) |
Case Studies
- Study on Tesaglitazar : A dual PPARα/γ agonist demonstrated improvements in insulin sensitivity and lipid profiles in patients with T2D over a one-year treatment period. Patients exhibited significant reductions in liver steatosis and inflammation .
- Long-term Effects : In a cohort study involving patients treated with pioglitazone (a PPARγ agonist), significant improvements were noted in glycemic control and reduction of cardiovascular risk factors after one year .
Safety Profile
While PPAR agonists provide substantial benefits, they are also associated with certain adverse effects. Notably, some compounds have been linked to weight gain and fluid retention due to their action on adipose tissue . Recent studies emphasize the importance of selective modulation to minimize these side effects while retaining therapeutic efficacy.
Table 2: Adverse Effects Associated with PPAR Agonists
Adverse Effect | Frequency | Comments |
---|---|---|
Weight Gain | Common | Particularly with full agonists |
Edema | Moderate | Observed with certain dual agonists |
Cardiovascular Events | Rare | Risk associated with specific agents |
Future Directions
The development of selective PPAR modulators aims to enhance therapeutic efficacy while reducing side effects associated with traditional full agonists. Research continues into identifying compounds that can selectively activate specific PPAR subtypes or modulate their activity through different cofactor interactions .
Propiedades
IUPAC Name |
(2S)-2-methoxy-3-[4-[3-(4-methylsulfonyloxyphenyl)propylamino]phenyl]propanoic acid | |
---|---|---|
Source | PubChem | |
URL | https://pubchem.ncbi.nlm.nih.gov | |
Description | Data deposited in or computed by PubChem | |
InChI |
InChI=1S/C20H25NO6S/c1-26-19(20(22)23)14-16-5-9-17(10-6-16)21-13-3-4-15-7-11-18(12-8-15)27-28(2,24)25/h5-12,19,21H,3-4,13-14H2,1-2H3,(H,22,23)/t19-/m0/s1 | |
Source | PubChem | |
URL | https://pubchem.ncbi.nlm.nih.gov | |
Description | Data deposited in or computed by PubChem | |
InChI Key |
HFRCAKXUSHNVLY-IBGZPJMESA-N | |
Source | PubChem | |
URL | https://pubchem.ncbi.nlm.nih.gov | |
Description | Data deposited in or computed by PubChem | |
Canonical SMILES |
COC(CC1=CC=C(C=C1)NCCCC2=CC=C(C=C2)OS(=O)(=O)C)C(=O)O | |
Source | PubChem | |
URL | https://pubchem.ncbi.nlm.nih.gov | |
Description | Data deposited in or computed by PubChem | |
Isomeric SMILES |
CO[C@@H](CC1=CC=C(C=C1)NCCCC2=CC=C(C=C2)OS(=O)(=O)C)C(=O)O | |
Source | PubChem | |
URL | https://pubchem.ncbi.nlm.nih.gov | |
Description | Data deposited in or computed by PubChem | |
Molecular Formula |
C20H25NO6S | |
Source | PubChem | |
URL | https://pubchem.ncbi.nlm.nih.gov | |
Description | Data deposited in or computed by PubChem | |
Molecular Weight |
407.5 g/mol | |
Source | PubChem | |
URL | https://pubchem.ncbi.nlm.nih.gov | |
Description | Data deposited in or computed by PubChem | |
Retrosynthesis Analysis
AI-Powered Synthesis Planning: Our tool employs the Template_relevance Pistachio, Template_relevance Bkms_metabolic, Template_relevance Pistachio_ringbreaker, Template_relevance Reaxys, Template_relevance Reaxys_biocatalysis model, leveraging a vast database of chemical reactions to predict feasible synthetic routes.
One-Step Synthesis Focus: Specifically designed for one-step synthesis, it provides concise and direct routes for your target compounds, streamlining the synthesis process.
Accurate Predictions: Utilizing the extensive PISTACHIO, BKMS_METABOLIC, PISTACHIO_RINGBREAKER, REAXYS, REAXYS_BIOCATALYSIS database, our tool offers high-accuracy predictions, reflecting the latest in chemical research and data.
Strategy Settings
Precursor scoring | Relevance Heuristic |
---|---|
Min. plausibility | 0.01 |
Model | Template_relevance |
Template Set | Pistachio/Bkms_metabolic/Pistachio_ringbreaker/Reaxys/Reaxys_biocatalysis |
Top-N result to add to graph | 6 |
Feasible Synthetic Routes
Descargo de responsabilidad e información sobre productos de investigación in vitro
Tenga en cuenta que todos los artículos e información de productos presentados en BenchChem están destinados únicamente con fines informativos. Los productos disponibles para la compra en BenchChem están diseñados específicamente para estudios in vitro, que se realizan fuera de organismos vivos. Los estudios in vitro, derivados del término latino "in vidrio", involucran experimentos realizados en entornos de laboratorio controlados utilizando células o tejidos. Es importante tener en cuenta que estos productos no se clasifican como medicamentos y no han recibido la aprobación de la FDA para la prevención, tratamiento o cura de ninguna condición médica, dolencia o enfermedad. Debemos enfatizar que cualquier forma de introducción corporal de estos productos en humanos o animales está estrictamente prohibida por ley. Es esencial adherirse a estas pautas para garantizar el cumplimiento de los estándares legales y éticos en la investigación y experimentación.