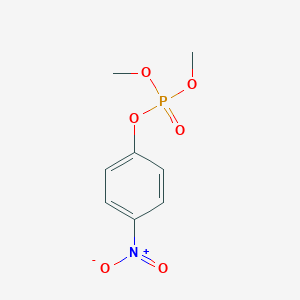
Metil paraoxón
Descripción general
Descripción
Paraoxon-methyl is an oxon derivative and a major metabolite of parathionbelonging to the class of organophosphate insecticides.
Paraoxon-methyl is a C-nitro compound.
Aplicaciones Científicas De Investigación
Toxicología ambiental
El metil paraoxón (MP) es un plaguicida de uso restringido que se ha utilizado ampliamente como insecticida agrícola . Pertenece a la clase de productos químicos organofosforados que se caracterizan por su capacidad para inhibir la actividad de la acetilcolinesterasa . La principal vía de exposición humana es la inhalación, pero el contacto dérmico y la ingestión accidental también pueden ser sustanciales .
Efectos sobre la salud
La exposición a MP puede provocar efectos sobre la salud, como dolores de cabeza, náuseas, despertares nocturnos, diarrea, dificultad para respirar, sudoración y salivación excesivas, incoordinación y confusión mental . También se han reportado otros síntomas, como problemas de comportamiento, problemas de habilidades motoras y deterioro del recuerdo .
Aplicaciones agrícolas
El this compound se aplica a varios cultivos como alfalfa, cebada, maíz, algodón, sorgo, soya, girasol y trigo . Se utiliza para controlar el picudo del algodón y muchos insectos picadores de los cultivos agrícolas, principalmente el algodón .
Neurotoxicidad
El this compound, el metabolito activo del metil paratión, inactiva la acetilcolinesterasa al fosforilar el sitio activo de la enzima . Esta acción inhibe la hidrólisis de la acetilcolina y el neurotransmisor se acumula en el sitio de acción, lo que produce una sobreestimulación de los órganos terminales colinérgicos .
Métodos de detección
Muchos métodos de detección para MP se basan en el efecto catalítico o de inhibición de la enzima
Mecanismo De Acción
Target of Action
Methyl paraoxon (MP) is a highly toxic organophosphorus pesticide . Its primary target is acetylcholinesterase (AChE) , an enzyme crucial for the proper functioning of the nervous system . AChE’s role is to break down acetylcholine, a neurotransmitter, in the synaptic cleft, thereby terminating the signal transmission .
Mode of Action
Methyl paraoxon acts by inhibiting the activity of AChE . Specifically, it inactivates AChE by phosphorylating the active site of the enzyme . This action prevents the hydrolysis of acetylcholine, leading to its accumulation at the site of action . The result is an over-stimulation of cholinergic end organs .
Biochemical Pathways
The inhibition of AChE disrupts the normal functioning of the nervous system. The accumulation of acetylcholine leads to continuous stimulation of the neurons, causing a range of symptoms including headaches, nausea, difficulty breathing, excessive sweating and salivation, incoordination, and mental confusion .
Pharmacokinetics
The pharmacokinetics of methyl parathion, the parent compound of methyl paraoxon, have been studied . After intravenous administration, it was found to follow a three-compartment model . The apparent volume of the central compartment was 1.45 liters/kg, clearance was 1.85 liters/h/kg, and the terminal half-life was 6.6 h with an elimination constant of 0.50 h−1 .
Result of Action
The inhibition of AChE by methyl paraoxon leads to a range of health effects. These include symptoms related to the nervous system such as headaches, muscle weakness, insomnia, dizziness, and impaired memory . Other effects include cardiovascular lesions, abnormalities in heart rate, and an increase in the heart-to-body ratio . Reproductive effects such as changes in placental morphology, fibrosis and hemorrhage, and inhibition of DNA synthesis in seminiferous tubules have also been reported .
Action Environment
The degradation of organophosphates like methyl paraoxon is influenced by environmental factors such as pH, microbial activity, and light exposure . Hydrolysis, which is a significant degradation pathway, mainly occurs at pH >7 . Microbial degradation and photolysis also contribute to the breakdown of these compounds .
Análisis Bioquímico
Biochemical Properties
Methyl paraoxon is characterized by its ability to inhibit acetylcholinesterase activity . The active metabolite of methyl parathion, methyl paraoxon, inactivates acetylcholinesterase by phosphorylating the active site of the enzyme . This action inhibits the hydrolysis of acetylcholine, leading to an accumulation of the neurotransmitter at the site of action, thereby producing over-stimulation of cholinergic end organs .
Cellular Effects
Methyl paraoxon has been shown to have cytotoxic effects on a mouse neuroblastoma cell line NB41A3 . It leads to apoptosis and reduced cell replication in differentiated PC12 cells taken from rat adrenal medulla pheochromocytoma . It also causes symptoms such as headaches, nausea, night-waking, diarrhea, difficulty breathing, excessive sweating and salivation, incoordination, and mental confusion .
Molecular Mechanism
The primary mechanism of toxicity of methyl paraoxon is the inhibition of acetylcholinesterase activity in the nervous system and at the motor end-plate . Methyl paraoxon, the active metabolite of methyl parathion, inactivates acetylcholinesterase by phosphorylating the active site of the enzyme .
Temporal Effects in Laboratory Settings
The effects of methyl paraoxon have been studied over time in laboratory settings
Dosage Effects in Animal Models
In animal models, the effects of methyl paraoxon vary with different dosages
Metabolic Pathways
Methyl paraoxon is involved in metabolic pathways that inhibit acetylcholinesterase activity
Propiedades
IUPAC Name |
dimethyl (4-nitrophenyl) phosphate | |
---|---|---|
Source | PubChem | |
URL | https://pubchem.ncbi.nlm.nih.gov | |
Description | Data deposited in or computed by PubChem | |
InChI |
InChI=1S/C8H10NO6P/c1-13-16(12,14-2)15-8-5-3-7(4-6-8)9(10)11/h3-6H,1-2H3 | |
Source | PubChem | |
URL | https://pubchem.ncbi.nlm.nih.gov | |
Description | Data deposited in or computed by PubChem | |
InChI Key |
BAFQDKPJKOLXFZ-UHFFFAOYSA-N | |
Source | PubChem | |
URL | https://pubchem.ncbi.nlm.nih.gov | |
Description | Data deposited in or computed by PubChem | |
Canonical SMILES |
COP(=O)(OC)OC1=CC=C(C=C1)[N+](=O)[O-] | |
Source | PubChem | |
URL | https://pubchem.ncbi.nlm.nih.gov | |
Description | Data deposited in or computed by PubChem | |
Molecular Formula |
C8H10NO6P | |
Source | PubChem | |
URL | https://pubchem.ncbi.nlm.nih.gov | |
Description | Data deposited in or computed by PubChem | |
DSSTOX Substance ID |
DTXSID5037571 | |
Record name | Methylparaoxon | |
Source | EPA DSSTox | |
URL | https://comptox.epa.gov/dashboard/DTXSID5037571 | |
Description | DSSTox provides a high quality public chemistry resource for supporting improved predictive toxicology. | |
Molecular Weight |
247.14 g/mol | |
Source | PubChem | |
URL | https://pubchem.ncbi.nlm.nih.gov | |
Description | Data deposited in or computed by PubChem | |
CAS No. |
950-35-6 | |
Record name | Paraoxon methyl | |
Source | CAS Common Chemistry | |
URL | https://commonchemistry.cas.org/detail?cas_rn=950-35-6 | |
Description | CAS Common Chemistry is an open community resource for accessing chemical information. Nearly 500,000 chemical substances from CAS REGISTRY cover areas of community interest, including common and frequently regulated chemicals, and those relevant to high school and undergraduate chemistry classes. This chemical information, curated by our expert scientists, is provided in alignment with our mission as a division of the American Chemical Society. | |
Explanation | The data from CAS Common Chemistry is provided under a CC-BY-NC 4.0 license, unless otherwise stated. | |
Record name | Methylparaoxon | |
Source | ChemIDplus | |
URL | https://pubchem.ncbi.nlm.nih.gov/substance/?source=chemidplus&sourceid=0000950356 | |
Description | ChemIDplus is a free, web search system that provides access to the structure and nomenclature authority files used for the identification of chemical substances cited in National Library of Medicine (NLM) databases, including the TOXNET system. | |
Record name | Methyl paroxon | |
Source | EPA Acute Exposure Guideline Levels (AEGLs) | |
URL | https://www.epa.gov/aegl/access-acute-exposure-guideline-levels-aegls-values#tab-4 | |
Description | Acute Exposure Guideline Levels (AEGLs) are used by emergency planners and responders worldwide as guidance in dealing with rare, usually accidental, releases of chemicals into the air. https://www.epa.gov/aegl | |
Record name | Methylparaoxon | |
Source | EPA DSSTox | |
URL | https://comptox.epa.gov/dashboard/DTXSID5037571 | |
Description | DSSTox provides a high quality public chemistry resource for supporting improved predictive toxicology. | |
Record name | Methylparaoxon | |
Source | European Chemicals Agency (ECHA) | |
URL | https://echa.europa.eu/information-on-chemicals | |
Description | The European Chemicals Agency (ECHA) is an agency of the European Union which is the driving force among regulatory authorities in implementing the EU's groundbreaking chemicals legislation for the benefit of human health and the environment as well as for innovation and competitiveness. | |
Explanation | Use of the information, documents and data from the ECHA website is subject to the terms and conditions of this Legal Notice, and subject to other binding limitations provided for under applicable law, the information, documents and data made available on the ECHA website may be reproduced, distributed and/or used, totally or in part, for non-commercial purposes provided that ECHA is acknowledged as the source: "Source: European Chemicals Agency, http://echa.europa.eu/". Such acknowledgement must be included in each copy of the material. ECHA permits and encourages organisations and individuals to create links to the ECHA website under the following cumulative conditions: Links can only be made to webpages that provide a link to the Legal Notice page. | |
Record name | METHYL PARAOXON | |
Source | FDA Global Substance Registration System (GSRS) | |
URL | https://gsrs.ncats.nih.gov/ginas/app/beta/substances/UE1A2XL95H | |
Description | The FDA Global Substance Registration System (GSRS) enables the efficient and accurate exchange of information on what substances are in regulated products. Instead of relying on names, which vary across regulatory domains, countries, and regions, the GSRS knowledge base makes it possible for substances to be defined by standardized, scientific descriptions. | |
Explanation | Unless otherwise noted, the contents of the FDA website (www.fda.gov), both text and graphics, are not copyrighted. They are in the public domain and may be republished, reprinted and otherwise used freely by anyone without the need to obtain permission from FDA. Credit to the U.S. Food and Drug Administration as the source is appreciated but not required. | |
Retrosynthesis Analysis
AI-Powered Synthesis Planning: Our tool employs the Template_relevance Pistachio, Template_relevance Bkms_metabolic, Template_relevance Pistachio_ringbreaker, Template_relevance Reaxys, Template_relevance Reaxys_biocatalysis model, leveraging a vast database of chemical reactions to predict feasible synthetic routes.
One-Step Synthesis Focus: Specifically designed for one-step synthesis, it provides concise and direct routes for your target compounds, streamlining the synthesis process.
Accurate Predictions: Utilizing the extensive PISTACHIO, BKMS_METABOLIC, PISTACHIO_RINGBREAKER, REAXYS, REAXYS_BIOCATALYSIS database, our tool offers high-accuracy predictions, reflecting the latest in chemical research and data.
Strategy Settings
Precursor scoring | Relevance Heuristic |
---|---|
Min. plausibility | 0.01 |
Model | Template_relevance |
Template Set | Pistachio/Bkms_metabolic/Pistachio_ringbreaker/Reaxys/Reaxys_biocatalysis |
Top-N result to add to graph | 6 |
Feasible Synthetic Routes
Q1: What is the primary mechanism of toxicity for methyl paraoxon?
A1: Methyl paraoxon exerts its toxicity by inhibiting acetylcholinesterase (AChE), a critical enzyme responsible for hydrolyzing the neurotransmitter acetylcholine (ACh) [, , ].
Q2: What are the consequences of acetylcholinesterase inhibition by methyl paraoxon?
A2: Inhibition of AChE leads to an accumulation of ACh in the synaptic cleft, causing excessive stimulation of cholinergic receptors and a range of symptoms associated with cholinergic excess [, , ].
Q3: Does the route of methyl paraoxon exposure influence its effects on cholinesterase activity?
A3: Yes, research indicates significant differences in cholinesterase inhibition patterns depending on the route of methyl paraoxon exposure. Intravenous and oral administration result in rapid but transient inhibition, whereas dermal exposure leads to a slower and more prolonged inhibition, suggesting route-dependent differences in absorption, distribution, metabolism, and/or excretion [].
Q4: Do different tissues exhibit varying sensitivities to methyl paraoxon-induced cholinesterase inhibition?
A4: Yes, studies show that brain regions may exhibit varying sensitivities to methyl paraoxon. For instance, the brain stem appears highly sensitive to inhibition of both AChE activity and muscarinic receptor binding by methyl paraoxon, potentially contributing to the lethal toxicity of this organophosphate [].
Q5: Can methyl paraoxon affect other enzymes besides acetylcholinesterase?
A5: Yes, research has shown that methyl paraoxon can inhibit other esterases, particularly aliesterases, which play a role in detoxifying organophosphates. The balance between AChE inhibition and aliesterase activity contributes to the overall toxicity of methyl paraoxon [, ].
Q6: What is the molecular formula and weight of methyl paraoxon?
A6: The molecular formula of methyl paraoxon is C8H10NO6P, and its molecular weight is 247.15 g/mol.
Q7: What approaches are being explored for the degradation of methyl paraoxon?
A7: Several strategies are under investigation for methyl paraoxon degradation, including:* Catalytic degradation: Materials like metal-organic frameworks (MOFs) [, , , ] and metal oxides [, ] have shown promise in catalyzing the hydrolysis of methyl paraoxon.* Enzymatic degradation: Organophosphate hydrolase (OPH) can break down methyl paraoxon. Research is ongoing to enhance OPH stability and activity in various environments, including using complex coacervate core micelles [] and immobilizing it on carbon nanotube paper [].
Q8: How does moisture affect the degradation of methyl paraoxon by metal-organic frameworks?
A8: Research suggests that the presence of moisture significantly influences the hydrolytic activity of certain Zr-based MOFs, like UiO-66, towards methyl paraoxon. Increasing water content in the MOF structure enhances its degradation rate, highlighting the importance of moisture in solid-phase decontamination [].
Q9: How do metal-organic frameworks (MOFs) catalyze the degradation of methyl paraoxon?
A9: Zr-based MOFs, like UiO-66, possess Lewis acidic sites (Zr⁴⁺) that can interact with the phosphoryl oxygen of methyl paraoxon, facilitating nucleophilic attack by water molecules and subsequent hydrolysis [].
Q10: What factors influence the catalytic efficiency of MOFs in degrading methyl paraoxon?
A10: Factors like the type of organic linker, pore size, and accessibility of active sites in the MOF structure play a role in determining its catalytic efficiency for methyl paraoxon hydrolysis.
Q11: Have computational methods been used to study methyl paraoxon degradation?
A11: Yes, density functional theory (DFT) calculations have been employed to study the adsorption energies of methyl paraoxon and other molecules on catalyst surfaces, providing insights into the degradation mechanism [].
Q12: How does the structure of methyl paraoxon contribute to its toxicity compared to its parent compound, methyl parathion?
A12: The P=O bond in methyl paraoxon makes it a more potent inhibitor of AChE compared to its P=S counterpart, methyl parathion. This is because the P=O bond is more electrophilic and readily forms a stable covalent bond with the active site serine of AChE [, ].
Q13: Are there specific safety concerns associated with handling methyl paraoxon in a research setting?
A13: Yes, due to its high toxicity, methyl paraoxon should be handled with extreme caution in a well-ventilated fume hood using appropriate personal protective equipment, including gloves, lab coat, and eye protection. Adherence to established safety protocols and regulations is crucial when working with this compound.
Q14: How does the uptake of methyl paraoxon differ from its parent compound, methyl parathion, in cellular systems?
A15: Studies using radiolabeled compounds demonstrated a higher uptake of methyl paraoxon in SH-SY5Y cells compared to methyl parathion. This difference in uptake contributes to the higher cytotoxicity observed with methyl paraoxon [].
Q15: What types of in vitro models are used to study methyl paraoxon toxicity?
A16: Researchers utilize various in vitro models, including:* Enzyme assays: These assess the inhibitory potency of methyl paraoxon on AChE and other esterases [, , ].* Cell lines: Cultures of mammalian cells, such as SH-SY5Y cells, are used to investigate the cellular uptake, metabolism, and cytotoxic effects of methyl paraoxon [].
Q16: Have resistance mechanisms to methyl paraoxon been observed in insects?
A17: Yes, studies on house fly larvae resistant to methyl parathion have revealed two primary resistance mechanisms: enhanced metabolic detoxification of methyl paraoxon and decreased target site sensitivity due to structural changes in AChE [].
Q17: What are the acute toxic effects of methyl paraoxon in mammals?
A18: Methyl paraoxon, being a potent AChE inhibitor, can cause a range of cholinergic symptoms in mammals, including:* Muscarinic effects: Salivation, lacrimation, urination, defecation, gastrointestinal cramps, and bronchoconstriction.* Nicotinic effects: Muscle twitching, weakness, paralysis, and respiratory failure.* Central nervous system effects: Anxiety, confusion, seizures, coma, and respiratory arrest [, ].
Q18: What analytical techniques are commonly used for the detection and quantification of methyl paraoxon?
A19: Common analytical methods include:* Gas chromatography (GC): Often coupled with electron capture (ECD) or flame photometric (FPD) detectors for sensitive and selective detection of methyl paraoxon in environmental and biological samples [, , ].* Liquid chromatography (LC): Frequently combined with tandem mass spectrometry (MS/MS) for precise identification and quantification of methyl paraoxon and its metabolites [, ].* Thin layer chromatography (TLC): A relatively simple and rapid technique used for qualitative analysis of methyl paraoxon, often based on cholinesterase inhibition assays [].* Electrochemical detection: Electrochemical methods using modified electrodes have also been explored for the detection of methyl paraoxon based on the electrochemical response of p-nitrophenol generated after hydrolysis [].
Q19: What are the potential environmental concerns associated with methyl paraoxon?
A20: As an organophosphate pesticide metabolite, methyl paraoxon raises concerns due to its:* Toxicity to non-target organisms: It can negatively impact beneficial insects, birds, aquatic life, and other organisms in the ecosystem [].* Persistence in the environment: Depending on environmental conditions, it can persist in soil and water, potentially leading to bioaccumulation and long-term ecological effects.
Descargo de responsabilidad e información sobre productos de investigación in vitro
Tenga en cuenta que todos los artículos e información de productos presentados en BenchChem están destinados únicamente con fines informativos. Los productos disponibles para la compra en BenchChem están diseñados específicamente para estudios in vitro, que se realizan fuera de organismos vivos. Los estudios in vitro, derivados del término latino "in vidrio", involucran experimentos realizados en entornos de laboratorio controlados utilizando células o tejidos. Es importante tener en cuenta que estos productos no se clasifican como medicamentos y no han recibido la aprobación de la FDA para la prevención, tratamiento o cura de ninguna condición médica, dolencia o enfermedad. Debemos enfatizar que cualquier forma de introducción corporal de estos productos en humanos o animales está estrictamente prohibida por ley. Es esencial adherirse a estas pautas para garantizar el cumplimiento de los estándares legales y éticos en la investigación y experimentación.