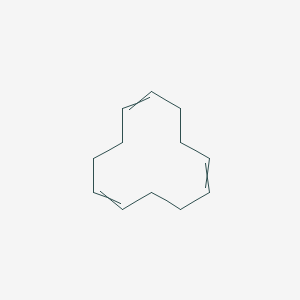
Cyclododeca-1,5,9-triene
Descripción general
Descripción
Cyclododeca-1,5,9-triene is a colorless liquid . It has a molecular formula of C12H18 . It is used to make other chemicals .
Synthesis Analysis
Cyclododeca-1,5,9-triene may react vigorously with strong oxidizing agents. It may react exothermically with reducing agents to release hydrogen gas . In the presence of various catalysts (such as acids) or initiators, it may undergo exothermic addition polymerization reactions . A study has shown that the interaction of cyclododeca-1,5,9-triene isomers with Ru3(CO)12 gives four complexes .Molecular Structure Analysis
The molecular structure of Cyclododeca-1,5,9-triene can be represented by the formula C12H18 . The structure is also available as a 2D Mol file or as a computed 3D SD file .Chemical Reactions Analysis
Cyclododeca-1,5,9-triene may react vigorously with strong oxidizing agents. It may react exothermically with reducing agents to release hydrogen gas . In the presence of various catalysts (such as acids) or initiators, it may undergo exothermic addition polymerization reactions .Physical And Chemical Properties Analysis
Cyclododeca-1,5,9-triene has a melting point of 33-35 °C (lit.), a boiling point of 237-238 °C (lit.), and a density of 0.89 g/mL at 20 °C (lit.) . Its vapor pressure is 12Pa at 20℃ and its refractive index is 1.5082 (estimate) .Aplicaciones Científicas De Investigación
- The trans, trans, cis-isomer of cyclododecatriene serves as a crucial precursor in the production of nylon-12 , a high-performance polymer used in various applications such as automotive components, medical devices, and specialty textiles .
- Further oxidation of this mixture using nitric acid produces dodecanedioic acid, which finds applications in the production of polyamides, adhesives, and coatings .
- Researchers have explored these odors and their potential applications in perfumery, flavoring, and sensory studies .
- These complexes have been studied for their catalytic properties and potential applications in organic synthesis .
Nylon-12 Production
Dodecanedioic Acid Synthesis
Terpene-Like Odor Studies
Transition Metal Complex Formation
Transannular Reactions and Isomerization
Other Potential Applications
Mecanismo De Acción
Target of Action
Cyclododeca-1,5,9-triene is a chemical compound that primarily targets the process of trimerization of butadiene . This process is technically the most important process giving access to medium-ring compounds .
Mode of Action
The compound interacts with its targets through a process known as selective trimerization . This process is catalyzed by TiCl4 and ethylaluminum sesquichloride . The interaction results in the formation of 1,5,9-cyclododecatriene .
Biochemical Pathways
The primary biochemical pathway affected by Cyclododeca-1,5,9-triene is the trimerization of butadiene . The downstream effects of this pathway include the production of laurolactam and polyamide-12, which are important specialty engineering plastics for automotive applications . It is also used for the production of macrocyclic musks and agrochemicals .
Pharmacokinetics
It’s known that the compound may react exothermically with reducing agents to release hydrogen gas . In the presence of various catalysts (such as acids) or initiators, it may undergo exothermic addition polymerization reactions .
Result of Action
The molecular and cellular effects of Cyclododeca-1,5,9-triene’s action primarily involve the formation of 1,5,9-cyclododecatriene . This compound is a key intermediate in the production of several important substances, including laurolactam, polyamide-12, macrocyclic musks, and certain agrochemicals .
Action Environment
The action, efficacy, and stability of Cyclododeca-1,5,9-triene can be influenced by various environmental factors. For instance, the presence of strong oxidizing agents can cause it to react vigorously . Additionally, the presence of catalysts or initiators can trigger exothermic addition polymerization reactions .
Safety and Hazards
Direcciones Futuras
Propiedades
IUPAC Name |
cyclododeca-1,5,9-triene | |
---|---|---|
Source | PubChem | |
URL | https://pubchem.ncbi.nlm.nih.gov | |
Description | Data deposited in or computed by PubChem | |
InChI |
InChI=1S/C12H18/c1-2-4-6-8-10-12-11-9-7-5-3-1/h1-2,7-10H,3-6,11-12H2 | |
Source | PubChem | |
URL | https://pubchem.ncbi.nlm.nih.gov | |
Description | Data deposited in or computed by PubChem | |
InChI Key |
ZOLLIQAKMYWTBR-UHFFFAOYSA-N | |
Source | PubChem | |
URL | https://pubchem.ncbi.nlm.nih.gov | |
Description | Data deposited in or computed by PubChem | |
Canonical SMILES |
C1CC=CCCC=CCCC=C1 | |
Source | PubChem | |
URL | https://pubchem.ncbi.nlm.nih.gov | |
Description | Data deposited in or computed by PubChem | |
Molecular Formula |
C12H18 | |
Record name | 1,5,9-CYCLODODECATRIENE | |
Source | CAMEO Chemicals | |
URL | https://cameochemicals.noaa.gov/chemical/3039 | |
Description | CAMEO Chemicals is a chemical database designed for people who are involved in hazardous material incident response and planning. CAMEO Chemicals contains a library with thousands of datasheets containing response-related information and recommendations for hazardous materials that are commonly transported, used, or stored in the United States. CAMEO Chemicals was developed by the National Oceanic and Atmospheric Administration's Office of Response and Restoration in partnership with the Environmental Protection Agency's Office of Emergency Management. | |
Explanation | CAMEO Chemicals and all other CAMEO products are available at no charge to those organizations and individuals (recipients) responsible for the safe handling of chemicals. However, some of the chemical data itself is subject to the copyright restrictions of the companies or organizations that provided the data. | |
Source | PubChem | |
URL | https://pubchem.ncbi.nlm.nih.gov | |
Description | Data deposited in or computed by PubChem | |
DSSTOX Substance ID |
DTXSID8027581 | |
Record name | 1,5,9-Cyclododecatriene | |
Source | EPA DSSTox | |
URL | https://comptox.epa.gov/dashboard/DTXSID8027581 | |
Description | DSSTox provides a high quality public chemistry resource for supporting improved predictive toxicology. | |
Molecular Weight |
162.27 g/mol | |
Source | PubChem | |
URL | https://pubchem.ncbi.nlm.nih.gov | |
Description | Data deposited in or computed by PubChem | |
Physical Description |
1,5,9-cyclododecatriene appears as a colorless liquid. Toxic by skin absorption and ingestion and irritating to skin and eyes. Used to make other chemicals., Colorless liquid; [CAMEO] Very faintly yellow clear liquid; [MSDSonline] | |
Record name | 1,5,9-CYCLODODECATRIENE | |
Source | CAMEO Chemicals | |
URL | https://cameochemicals.noaa.gov/chemical/3039 | |
Description | CAMEO Chemicals is a chemical database designed for people who are involved in hazardous material incident response and planning. CAMEO Chemicals contains a library with thousands of datasheets containing response-related information and recommendations for hazardous materials that are commonly transported, used, or stored in the United States. CAMEO Chemicals was developed by the National Oceanic and Atmospheric Administration's Office of Response and Restoration in partnership with the Environmental Protection Agency's Office of Emergency Management. | |
Explanation | CAMEO Chemicals and all other CAMEO products are available at no charge to those organizations and individuals (recipients) responsible for the safe handling of chemicals. However, some of the chemical data itself is subject to the copyright restrictions of the companies or organizations that provided the data. | |
Record name | 1,5,9-Cyclododecatriene | |
Source | Haz-Map, Information on Hazardous Chemicals and Occupational Diseases | |
URL | https://haz-map.com/Agents/2830 | |
Description | Haz-Map® is an occupational health database designed for health and safety professionals and for consumers seeking information about the adverse effects of workplace exposures to chemical and biological agents. | |
Explanation | Copyright (c) 2022 Haz-Map(R). All rights reserved. Unless otherwise indicated, all materials from Haz-Map are copyrighted by Haz-Map(R). No part of these materials, either text or image may be used for any purpose other than for personal use. Therefore, reproduction, modification, storage in a retrieval system or retransmission, in any form or by any means, electronic, mechanical or otherwise, for reasons other than personal use, is strictly prohibited without prior written permission. | |
Boiling Point |
447.8 °F at 760 mmHg (USCG, 1999), 240 °C at 101.3 kPa | |
Record name | 1,5,9-CYCLODODECATRIENE | |
Source | CAMEO Chemicals | |
URL | https://cameochemicals.noaa.gov/chemical/3039 | |
Description | CAMEO Chemicals is a chemical database designed for people who are involved in hazardous material incident response and planning. CAMEO Chemicals contains a library with thousands of datasheets containing response-related information and recommendations for hazardous materials that are commonly transported, used, or stored in the United States. CAMEO Chemicals was developed by the National Oceanic and Atmospheric Administration's Office of Response and Restoration in partnership with the Environmental Protection Agency's Office of Emergency Management. | |
Explanation | CAMEO Chemicals and all other CAMEO products are available at no charge to those organizations and individuals (recipients) responsible for the safe handling of chemicals. However, some of the chemical data itself is subject to the copyright restrictions of the companies or organizations that provided the data. | |
Record name | 1,5,9-CYCLODODECATRIENE | |
Source | Hazardous Substances Data Bank (HSDB) | |
URL | https://pubchem.ncbi.nlm.nih.gov/source/hsdb/6481 | |
Description | The Hazardous Substances Data Bank (HSDB) is a toxicology database that focuses on the toxicology of potentially hazardous chemicals. It provides information on human exposure, industrial hygiene, emergency handling procedures, environmental fate, regulatory requirements, nanomaterials, and related areas. The information in HSDB has been assessed by a Scientific Review Panel. | |
Flash Point |
160 °F (USCG, 1999), 71 °C, 88 °C closed cup | |
Record name | 1,5,9-CYCLODODECATRIENE | |
Source | CAMEO Chemicals | |
URL | https://cameochemicals.noaa.gov/chemical/3039 | |
Description | CAMEO Chemicals is a chemical database designed for people who are involved in hazardous material incident response and planning. CAMEO Chemicals contains a library with thousands of datasheets containing response-related information and recommendations for hazardous materials that are commonly transported, used, or stored in the United States. CAMEO Chemicals was developed by the National Oceanic and Atmospheric Administration's Office of Response and Restoration in partnership with the Environmental Protection Agency's Office of Emergency Management. | |
Explanation | CAMEO Chemicals and all other CAMEO products are available at no charge to those organizations and individuals (recipients) responsible for the safe handling of chemicals. However, some of the chemical data itself is subject to the copyright restrictions of the companies or organizations that provided the data. | |
Record name | 1,5,9-Cyclododecatriene | |
Source | Haz-Map, Information on Hazardous Chemicals and Occupational Diseases | |
URL | https://haz-map.com/Agents/2830 | |
Description | Haz-Map® is an occupational health database designed for health and safety professionals and for consumers seeking information about the adverse effects of workplace exposures to chemical and biological agents. | |
Explanation | Copyright (c) 2022 Haz-Map(R). All rights reserved. Unless otherwise indicated, all materials from Haz-Map are copyrighted by Haz-Map(R). No part of these materials, either text or image may be used for any purpose other than for personal use. Therefore, reproduction, modification, storage in a retrieval system or retransmission, in any form or by any means, electronic, mechanical or otherwise, for reasons other than personal use, is strictly prohibited without prior written permission. | |
Record name | 1,5,9-CYCLODODECATRIENE | |
Source | Hazardous Substances Data Bank (HSDB) | |
URL | https://pubchem.ncbi.nlm.nih.gov/source/hsdb/6481 | |
Description | The Hazardous Substances Data Bank (HSDB) is a toxicology database that focuses on the toxicology of potentially hazardous chemicals. It provides information on human exposure, industrial hygiene, emergency handling procedures, environmental fate, regulatory requirements, nanomaterials, and related areas. The information in HSDB has been assessed by a Scientific Review Panel. | |
Density |
0.8925 (USCG, 1999) - Less dense than water; will float, 0.89 at 20 °C/20 °C, Density: 0.84 g/cu cm at 100 °C | |
Record name | 1,5,9-CYCLODODECATRIENE | |
Source | CAMEO Chemicals | |
URL | https://cameochemicals.noaa.gov/chemical/3039 | |
Description | CAMEO Chemicals is a chemical database designed for people who are involved in hazardous material incident response and planning. CAMEO Chemicals contains a library with thousands of datasheets containing response-related information and recommendations for hazardous materials that are commonly transported, used, or stored in the United States. CAMEO Chemicals was developed by the National Oceanic and Atmospheric Administration's Office of Response and Restoration in partnership with the Environmental Protection Agency's Office of Emergency Management. | |
Explanation | CAMEO Chemicals and all other CAMEO products are available at no charge to those organizations and individuals (recipients) responsible for the safe handling of chemicals. However, some of the chemical data itself is subject to the copyright restrictions of the companies or organizations that provided the data. | |
Record name | 1,5,9-CYCLODODECATRIENE | |
Source | Hazardous Substances Data Bank (HSDB) | |
URL | https://pubchem.ncbi.nlm.nih.gov/source/hsdb/6481 | |
Description | The Hazardous Substances Data Bank (HSDB) is a toxicology database that focuses on the toxicology of potentially hazardous chemicals. It provides information on human exposure, industrial hygiene, emergency handling procedures, environmental fate, regulatory requirements, nanomaterials, and related areas. The information in HSDB has been assessed by a Scientific Review Panel. | |
Vapor Pressure |
0.08 [mmHg] | |
Record name | 1,5,9-Cyclododecatriene | |
Source | Haz-Map, Information on Hazardous Chemicals and Occupational Diseases | |
URL | https://haz-map.com/Agents/2830 | |
Description | Haz-Map® is an occupational health database designed for health and safety professionals and for consumers seeking information about the adverse effects of workplace exposures to chemical and biological agents. | |
Explanation | Copyright (c) 2022 Haz-Map(R). All rights reserved. Unless otherwise indicated, all materials from Haz-Map are copyrighted by Haz-Map(R). No part of these materials, either text or image may be used for any purpose other than for personal use. Therefore, reproduction, modification, storage in a retrieval system or retransmission, in any form or by any means, electronic, mechanical or otherwise, for reasons other than personal use, is strictly prohibited without prior written permission. | |
Product Name |
Cyclododeca-1,5,9-triene | |
Color/Form |
Colorless, Liquid | |
CAS RN |
4904-61-4, 2765-29-9, 676-22-2, 706-31-0 | |
Record name | 1,5,9-CYCLODODECATRIENE | |
Source | CAMEO Chemicals | |
URL | https://cameochemicals.noaa.gov/chemical/3039 | |
Description | CAMEO Chemicals is a chemical database designed for people who are involved in hazardous material incident response and planning. CAMEO Chemicals contains a library with thousands of datasheets containing response-related information and recommendations for hazardous materials that are commonly transported, used, or stored in the United States. CAMEO Chemicals was developed by the National Oceanic and Atmospheric Administration's Office of Response and Restoration in partnership with the Environmental Protection Agency's Office of Emergency Management. | |
Explanation | CAMEO Chemicals and all other CAMEO products are available at no charge to those organizations and individuals (recipients) responsible for the safe handling of chemicals. However, some of the chemical data itself is subject to the copyright restrictions of the companies or organizations that provided the data. | |
Record name | 1,5,9-Cyclododecatriene | |
Source | EPA Chemicals under the TSCA | |
URL | https://www.epa.gov/chemicals-under-tsca | |
Description | EPA Chemicals under the Toxic Substances Control Act (TSCA) collection contains information on chemicals and their regulations under TSCA, including non-confidential content from the TSCA Chemical Substance Inventory and Chemical Data Reporting. | |
Record name | 1,5,9-Cyclododecatriene | |
Source | EPA DSSTox | |
URL | https://comptox.epa.gov/dashboard/DTXSID8027581 | |
Description | DSSTox provides a high quality public chemistry resource for supporting improved predictive toxicology. | |
Record name | Cyclododeca-1,5,9-triene | |
Source | European Chemicals Agency (ECHA) | |
URL | https://echa.europa.eu/substance-information/-/substanceinfo/100.023.212 | |
Description | The European Chemicals Agency (ECHA) is an agency of the European Union which is the driving force among regulatory authorities in implementing the EU's groundbreaking chemicals legislation for the benefit of human health and the environment as well as for innovation and competitiveness. | |
Explanation | Use of the information, documents and data from the ECHA website is subject to the terms and conditions of this Legal Notice, and subject to other binding limitations provided for under applicable law, the information, documents and data made available on the ECHA website may be reproduced, distributed and/or used, totally or in part, for non-commercial purposes provided that ECHA is acknowledged as the source: "Source: European Chemicals Agency, http://echa.europa.eu/". Such acknowledgement must be included in each copy of the material. ECHA permits and encourages organisations and individuals to create links to the ECHA website under the following cumulative conditions: Links can only be made to webpages that provide a link to the Legal Notice page. | |
Record name | trans,cis,cis-cyclododeca-1,5,9-triene | |
Source | European Chemicals Agency (ECHA) | |
URL | https://echa.europa.eu/substance-information/-/substanceinfo/100.018.580 | |
Description | The European Chemicals Agency (ECHA) is an agency of the European Union which is the driving force among regulatory authorities in implementing the EU's groundbreaking chemicals legislation for the benefit of human health and the environment as well as for innovation and competitiveness. | |
Explanation | Use of the information, documents and data from the ECHA website is subject to the terms and conditions of this Legal Notice, and subject to other binding limitations provided for under applicable law, the information, documents and data made available on the ECHA website may be reproduced, distributed and/or used, totally or in part, for non-commercial purposes provided that ECHA is acknowledged as the source: "Source: European Chemicals Agency, http://echa.europa.eu/". Such acknowledgement must be included in each copy of the material. ECHA permits and encourages organisations and individuals to create links to the ECHA website under the following cumulative conditions: Links can only be made to webpages that provide a link to the Legal Notice page. | |
Record name | (1E,5E,9E)-cyclododeca-1,5,9-triene | |
Source | European Chemicals Agency (ECHA) | |
URL | https://echa.europa.eu/substance-information/-/substanceinfo/100.010.570 | |
Description | The European Chemicals Agency (ECHA) is an agency of the European Union which is the driving force among regulatory authorities in implementing the EU's groundbreaking chemicals legislation for the benefit of human health and the environment as well as for innovation and competitiveness. | |
Explanation | Use of the information, documents and data from the ECHA website is subject to the terms and conditions of this Legal Notice, and subject to other binding limitations provided for under applicable law, the information, documents and data made available on the ECHA website may be reproduced, distributed and/or used, totally or in part, for non-commercial purposes provided that ECHA is acknowledged as the source: "Source: European Chemicals Agency, http://echa.europa.eu/". Such acknowledgement must be included in each copy of the material. ECHA permits and encourages organisations and individuals to create links to the ECHA website under the following cumulative conditions: Links can only be made to webpages that provide a link to the Legal Notice page. | |
Record name | (1E,5E,9Z)-cyclododeca-1,5,9-triene | |
Source | European Chemicals Agency (ECHA) | |
URL | https://echa.europa.eu/substance-information/-/substanceinfo/100.010.814 | |
Description | The European Chemicals Agency (ECHA) is an agency of the European Union which is the driving force among regulatory authorities in implementing the EU's groundbreaking chemicals legislation for the benefit of human health and the environment as well as for innovation and competitiveness. | |
Explanation | Use of the information, documents and data from the ECHA website is subject to the terms and conditions of this Legal Notice, and subject to other binding limitations provided for under applicable law, the information, documents and data made available on the ECHA website may be reproduced, distributed and/or used, totally or in part, for non-commercial purposes provided that ECHA is acknowledged as the source: "Source: European Chemicals Agency, http://echa.europa.eu/". Such acknowledgement must be included in each copy of the material. ECHA permits and encourages organisations and individuals to create links to the ECHA website under the following cumulative conditions: Links can only be made to webpages that provide a link to the Legal Notice page. | |
Record name | 1,5,9-CYCLODODECATRIENE | |
Source | Hazardous Substances Data Bank (HSDB) | |
URL | https://pubchem.ncbi.nlm.nih.gov/source/hsdb/6481 | |
Description | The Hazardous Substances Data Bank (HSDB) is a toxicology database that focuses on the toxicology of potentially hazardous chemicals. It provides information on human exposure, industrial hygiene, emergency handling procedures, environmental fate, regulatory requirements, nanomaterials, and related areas. The information in HSDB has been assessed by a Scientific Review Panel. | |
Melting Point |
-0.4 °F (USCG, 1999), -17 °C | |
Record name | 1,5,9-CYCLODODECATRIENE | |
Source | CAMEO Chemicals | |
URL | https://cameochemicals.noaa.gov/chemical/3039 | |
Description | CAMEO Chemicals is a chemical database designed for people who are involved in hazardous material incident response and planning. CAMEO Chemicals contains a library with thousands of datasheets containing response-related information and recommendations for hazardous materials that are commonly transported, used, or stored in the United States. CAMEO Chemicals was developed by the National Oceanic and Atmospheric Administration's Office of Response and Restoration in partnership with the Environmental Protection Agency's Office of Emergency Management. | |
Explanation | CAMEO Chemicals and all other CAMEO products are available at no charge to those organizations and individuals (recipients) responsible for the safe handling of chemicals. However, some of the chemical data itself is subject to the copyright restrictions of the companies or organizations that provided the data. | |
Record name | 1,5,9-CYCLODODECATRIENE | |
Source | Hazardous Substances Data Bank (HSDB) | |
URL | https://pubchem.ncbi.nlm.nih.gov/source/hsdb/6481 | |
Description | The Hazardous Substances Data Bank (HSDB) is a toxicology database that focuses on the toxicology of potentially hazardous chemicals. It provides information on human exposure, industrial hygiene, emergency handling procedures, environmental fate, regulatory requirements, nanomaterials, and related areas. The information in HSDB has been assessed by a Scientific Review Panel. | |
Synthesis routes and methods
Procedure details
Retrosynthesis Analysis
AI-Powered Synthesis Planning: Our tool employs the Template_relevance Pistachio, Template_relevance Bkms_metabolic, Template_relevance Pistachio_ringbreaker, Template_relevance Reaxys, Template_relevance Reaxys_biocatalysis model, leveraging a vast database of chemical reactions to predict feasible synthetic routes.
One-Step Synthesis Focus: Specifically designed for one-step synthesis, it provides concise and direct routes for your target compounds, streamlining the synthesis process.
Accurate Predictions: Utilizing the extensive PISTACHIO, BKMS_METABOLIC, PISTACHIO_RINGBREAKER, REAXYS, REAXYS_BIOCATALYSIS database, our tool offers high-accuracy predictions, reflecting the latest in chemical research and data.
Strategy Settings
Precursor scoring | Relevance Heuristic |
---|---|
Min. plausibility | 0.01 |
Model | Template_relevance |
Template Set | Pistachio/Bkms_metabolic/Pistachio_ringbreaker/Reaxys/Reaxys_biocatalysis |
Top-N result to add to graph | 6 |
Feasible Synthetic Routes
Descargo de responsabilidad e información sobre productos de investigación in vitro
Tenga en cuenta que todos los artículos e información de productos presentados en BenchChem están destinados únicamente con fines informativos. Los productos disponibles para la compra en BenchChem están diseñados específicamente para estudios in vitro, que se realizan fuera de organismos vivos. Los estudios in vitro, derivados del término latino "in vidrio", involucran experimentos realizados en entornos de laboratorio controlados utilizando células o tejidos. Es importante tener en cuenta que estos productos no se clasifican como medicamentos y no han recibido la aprobación de la FDA para la prevención, tratamiento o cura de ninguna condición médica, dolencia o enfermedad. Debemos enfatizar que cualquier forma de introducción corporal de estos productos en humanos o animales está estrictamente prohibida por ley. Es esencial adherirse a estas pautas para garantizar el cumplimiento de los estándares legales y éticos en la investigación y experimentación.