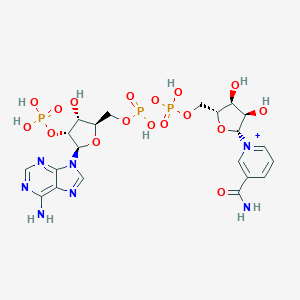
N-phenylacetyl-L-Homoserine lactone
Descripción general
Descripción
N-Phenylacetyl-L-homoserine lactone (N-phenylacetyl-L-HSL) is a synthetic quorum sensing (QS) signal molecule with a phenylacetyl group attached to the homoserine lactone core. Unlike natural acyl-homoserine lactones (AHLs) that typically feature aliphatic or oxo-substituted acyl chains, the aromatic phenyl group in N-phenylacetyl-L-HSL confers unique structural and functional properties. This compound has been shown to attenuate QS in Acinetobacter baumannii, a multidrug-resistant pathogen, by interfering with bacterial communication pathways .
Métodos De Preparación
Rutas sintéticas y condiciones de reacción: La N-fenilacetil-L-homoserina lactona se puede sintetizar a través de una serie de reacciones químicas que involucran la acilación de la L-homoserina lactona con ácido fenilacético. La reacción generalmente requiere un agente de acoplamiento como la diciclohexilcarbodiimida (DCC) y un catalizador como la 4-dimetilaminopiridina (DMAP) para facilitar la formación del enlace amida .
Métodos de producción industrial: La producción industrial de N-fenilacetil-L-homoserina lactona puede implicar métodos de bioconversión utilizando cepas de Escherichia coli modificadas genéticamente. Estas cepas están diseñadas para expresar enzimas específicas que catalizan la biosíntesis del compuesto a partir de moléculas precursoras .
Análisis De Reacciones Químicas
Tipos de reacciones: La N-fenilacetil-L-homoserina lactona sufre diversas reacciones químicas, que incluyen:
Oxidación: El compuesto se puede oxidar para formar ácidos carboxílicos correspondientes.
Reducción: Las reacciones de reducción pueden convertir el anillo lactona en un diol.
Sustitución: El grupo fenilacetilo puede sufrir reacciones de sustitución electrofílica aromática.
Reactivos y condiciones comunes:
Oxidación: Reactivos como el permanganato de potasio (KMnO4) o el trióxido de cromo (CrO3) en condiciones ácidas.
Reducción: Reactivos como el hidruro de litio y aluminio (LiAlH4) o el borohidruro de sodio (NaBH4).
Sustitución: Electrófilos como el bromo (Br2) o el ácido nítrico (HNO3) en condiciones controladas.
Principales productos:
Oxidación: Derivados del ácido fenilacético.
Reducción: Derivados de diol.
Sustitución: Derivados de fenilacetilo halogenados o nitrados.
Aplicaciones Científicas De Investigación
Quorum Sensing Modulation
Quorum Sensing Mechanism
Quorum sensing is a process where bacteria communicate using signaling molecules to coordinate group behaviors based on population density. PHL acts as a signaling molecule that can either inhibit or enhance QS pathways in various bacterial species, such as Vibrio fischeri and Pseudomonas aeruginosa .
Antagonistic and Agonistic Activities
Research indicates that PHL can function as both an antagonist and an agonist of QS receptors. For instance, it has been shown to inhibit the activity of LuxR-type receptors in Agrobacterium tumefaciens, while also acting as a superagonist in V. fischeri, significantly enhancing bioluminescence compared to native autoinducers .
Synthesis and Structure-Activity Relationships
Synthesis Methods
PHL can be synthesized through various methods, including microwave-assisted solid-phase synthesis, which allows for high purity and yield suitable for biological experiments .
Structure-Activity Relationships (SAR)
Studies have demonstrated that minor modifications to the PHL structure can lead to significant changes in its biological activity. For example, derivatives such as 4-bromo-PHL exhibit potent antagonistic properties against LuxR homologues .
Compound Name | Activity Type | Bacterial Target |
---|---|---|
PHL | Superagonist | V. fischeri |
4-bromo-PHL | Antagonist | A. tumefaciens |
N-(3-nitro-phenylacetanoyl)-L-homoserine lactone | Superagonist | V. fischeri |
Therapeutic Potential
Inhibition of Pathogenicity
The ability of PHL to modulate QS suggests potential therapeutic applications in controlling bacterial infections. By inhibiting QS mechanisms, PHL could reduce virulence factors in pathogenic bacteria, making them less harmful . This strategy is particularly relevant for treating infections caused by opportunistic pathogens like Pseudomonas aeruginosa.
Eukaryotic Responses
Plant Interactions
Recent studies indicate that eukaryotes, including plants such as Arabidopsis thaliana, respond to AHLs like PHL. These responses can affect growth and development, suggesting that bacterial signaling molecules may play roles in plant-microbe interactions . Understanding these interactions could lead to advancements in agricultural practices by utilizing microbial signals to enhance plant growth.
Case Studies and Research Findings
Several case studies highlight the diverse applications of PHL:
- Study on QS Modulation: Researchers synthesized a focused library of PHL derivatives to evaluate their effects on QS in V. fischeri. The findings revealed that structural variations significantly influenced agonistic and antagonistic activities .
- Plant Growth Responses: A systematic analysis demonstrated that exposure to AHLs altered seedling growth in model plants, indicating a complex interaction between bacterial signals and plant biology .
Mecanismo De Acción
La N-fenilacetil-L-homoserina lactona ejerce sus efectos a través de la detección de quórum al unirse a proteínas receptoras específicas en las bacterias. Estos receptores, como TraR en Agrobacterium tumefaciens y LasR en Pseudomonas aeruginosa, regulan la expresión de genes involucrados en la virulencia, la formación de biopelículas y otros procesos celulares. La unión de la N-fenilacetil-L-homoserina lactona a estos receptores puede activar o inhibir la expresión genética, dependiendo de la especie bacteriana y el receptor específico .
Comparación Con Compuestos Similares
Structural and Functional Comparison with Similar Compounds
Structural Features
The biological activity of AHLs is highly dependent on the acyl chain’s length, saturation, and substituents. Below is a comparative analysis of key AHLs:
Compound | Acyl Group Structure | Key Structural Features |
---|---|---|
N-Phenylacetyl-L-HSL | Phenylacetyl (aromatic) | Aromatic ring; no aliphatic chain |
N-Hexanoyl-L-HSL (C6-HSL) | Hexanoyl (C6, saturated) | Short aliphatic chain; no substituents |
N-Decanoyl-L-HSL (C10-HSL) | Decanoyl (C10, saturated) | Long aliphatic chain; no substituents |
N-(3-Oxododecanoyl)-L-HSL | 3-Oxododecanoyl (C12, 3-oxo) | Long chain with ketone group at C3 |
N-Heptanoyl-L-HSL | Heptanoyl (C7, saturated) | Intermediate aliphatic chain |
N-(3-Oxohexanoyl)-DL-HSL | 3-Oxohexanoyl (C6, 3-oxo) | Short chain with ketone group at C3 |
Quorum Sensing Modulation
- N-Phenylacetyl-L-HSL : Disrupts QS in A. baumannii by competing with natural AHLs for receptor binding, reducing virulence gene expression .
- C6-HSL and C10-HSL : Act as agonists in Chromobacterium violaceum, inducing violacein production. C10-HSL exhibits higher antimicrobial activity (MIC = 32 µg/mL) compared to C6-HSL (MIC = 64 µg/mL) .
- 3-Oxododecanoyl-L-HSL (3OC12-HSL): A key QS signal in Pseudomonas aeruginosa, it also induces apoptosis in mammalian mast cells at high concentrations (100 µM), highlighting cross-kingdom effects .
- N-(3-Oxohexanoyl)-DL-HSL: Serves as a natural ligand for LuxR-type receptors in Vibrio fischeri, demonstrating the importance of oxo-substituents in receptor activation .
Antimicrobial and Virulence Effects
- N-Phenylacetyl-L-HSL : Reduces biofilm formation in A. baumannii without direct antibacterial activity .
- C10-HSL and HSL4 (2-hexadecynoyl): Exhibit broad-spectrum antimicrobial activity against Gram-negative bacteria, with HSL4 showing superior efficacy due to its unsaturated acyl chain .
- 3OC12-HSL : Triggers IL-4 and IL-6 release in mast cells, linking bacterial QS molecules to immune modulation .
Metabolic Stability
Actividad Biológica
N-phenylacetyl-L-homoserine lactone (PHL) is a synthetic analog of homoserine lactones, which are crucial signaling molecules in bacterial quorum sensing (QS). This compound has garnered attention due to its unique biological activities, particularly its ability to modulate QS in various bacterial species. This article provides a comprehensive overview of the biological activity of PHL, including its mechanisms of action, structure-activity relationships (SAR), and implications for microbial interactions and potential applications in biocontrol.
PHL functions primarily as a modulator of QS, a process that enables bacteria to regulate gene expression based on population density. It can act as both an agonist and antagonist depending on the specific bacterial context:
- Agonistic Activity : PHL has been identified as a potent agonist in Vibrio fischeri, significantly enhancing bioluminescence and demonstrating up to tenfold higher activity compared to native autoinducers like octanoyl-homoserine lactone (OHHL) .
- Antagonistic Activity : Conversely, PHL can inhibit QS in pathogens such as Acinetobacter baumannii, thereby attenuating virulence factors associated with biofilm formation and pathogenicity .
Structure-Activity Relationships (SAR)
Research indicates that minor modifications in the PHL structure can lead to substantial changes in its biological activity. For instance:
- The introduction of halogen substituents (e.g., bromo, chloro) on the phenyl ring significantly influences the ligand's efficacy as an agonist or antagonist. Specific substitutions have been shown to enhance inhibitory activity against LuxR homologues in various Gram-negative bacteria .
- A detailed SAR analysis revealed that compounds with different substituents could either inhibit or activate QS pathways, highlighting the delicate balance between structural features and biological outcomes .
Case Studies and Research Findings
Several studies have investigated the biological effects of PHL and its derivatives:
- Quorum Sensing Modulation : A study demonstrated that PHL could effectively modulate QS in Vibrio fischeri, with varying degrees of luminescence induction observed across different structural analogs . The findings suggest that PHL’s structural diversity offers a rich platform for developing new QS modulators.
- Impact on Plant-Microbe Interactions : Research has shown that AHLs, including PHL, can influence plant growth and development by altering root morphology and promoting beneficial interactions with rhizobacteria . For example, Arabidopsis thaliana exhibited significant changes in root elongation when exposed to different concentrations of AHLs.
- Inhibition of Pathogenic Biofilms : In another study, PHL was utilized to investigate its effects on biofilm formation by Pseudomonas aeruginosa. The results indicated that PHL could disrupt biofilm integrity, thereby reducing the bacterium's virulence .
Data Tables
The following table summarizes key findings related to the biological activity of this compound:
Q & A
Basic Research Questions
Q. How can researchers detect and quantify N-phenylacetyl-L-homoserine lactone (C12-AHL) in bacterial cultures?
Methodological Answer: Detection and quantification rely on chromatographic separation paired with biosensors or mass spectrometry.
- Thin-Layer Chromatography (TLC) : Use Agrobacterium tumefaciens reporters with lacZ fusions to visualize AHLs via β-galactosidase activity. Mobility patterns distinguish structural variants (e.g., 3-oxo vs. 3-unsubstituted chains) .
- Liquid Chromatography-Tandem Mass Spectrometry (LC-MS/MS) : Enables simultaneous quantification of multiple AHLs, including rare or novel derivatives, with high sensitivity (detection limits ~0.1 nM) .
- Enzymatic Assays : AHL synthases (e.g., PhzI, LasI) can be studied in vitro using S-adenosylmethionine (SAM) and acyl-acyl carrier protein (acyl-ACP) substrates, with product validation via HPLC or radiolabeling .
Q. What experimental approaches are used to synthesize this compound?
Methodological Answer: Synthesis typically involves coupling activated acyl groups (e.g., phenylacetyl chloride) to L-homoserine lactone. Key steps include:
- Chemical Synthesis : Acyl chloride or anhydride derivatives react with L-homoserine lactone under basic conditions (e.g., triethylamine). Purification via silica gel chromatography ensures >95% purity .
- Enzymatic Synthesis : Recombinant AHL synthases (e.g., LuxI homologs) catalyze acyl transfer from acyl-ACP or CoA-thioesters to SAM-derived homoserine lactone. This method preserves stereochemistry .
Q. How does this compound function in quorum sensing (QS)?
Methodological Answer: C12-AHL binds transcriptional regulators (e.g., LuxR-type proteins), triggering population-wide gene activation. Key experimental validations include:
- Reporter Strains : Engineered E. coli or P. aeruginosa with AHL-responsive promoters (e.g., lasB-gfp) quantify signal potency .
- Gene Knockouts : Disruption of luxI (AHL synthase) or luxR (receptor) abolishes QS-dependent phenotypes (e.g., biofilm formation, virulence) .
Advanced Research Questions
Q. How do structural modifications in acyl side chains influence the activity of this compound?
Methodological Answer: Acyl chain length and substituents dictate receptor specificity and potency:
- Comparative Structure-Activity Studies : AHL analogs with varied chain lengths (C4–C18), oxidation states (3-oxo, 3-hydroxy), or unsaturation are tested in reporter assays. For example, 3-oxo substitutions enhance LasR binding in P. aeruginosa, while long chains (≥C12) exhibit antagonist properties .
- Crystallography : LasR-C12-AHL co-crystal structures reveal hydrophobic interactions between the acyl chain and receptor pocket, explaining specificity .
Q. What methodologies are employed to study the degradation of this compound by environmental bacteria?
Methodological Answer: Degradation pathways are dissected using:
- Metabolic Tracing : Radiolabeled (³H or ¹⁴C) AHLs track ring cleavage and mineralization. Variovorax paradoxus hydrolyzes AHLs to homoserine and fatty acids, utilizing them as carbon/nitrogen sources .
- Enzymatic Profiling : Lactonases (e.g., AiiA) and acylases are purified via affinity chromatography, with activity measured via HPLC or colorimetric assays .
Q. How do researchers resolve contradictions in data regarding the role of this compound across bacterial species?
Methodological Answer: Discrepancies arise from species-specific regulatory networks or experimental conditions:
- Cross-Species Complementation : Heterologous expression of luxI/R systems in E. coli isolates context-dependent effects (e.g., P. fluorescens produces atypical 3-hydroxy-AHLs) .
- Dose-Response Curves : Quantify EC₅₀ values to differentiate agonistic vs. antagonistic roles. For instance, C12-AHL inhibits QS in Chromobacterium violaceum but activates it in P. aeruginosa .
Q. What advanced techniques are used to identify novel AHL derivatives beyond traditional methods?
Methodological Answer: Non-targeted metabolomics and synthetic biology enable discovery:
- High-Resolution Mass Spectrometry (HR-MS) : Untargeted LC-HRMS identifies novel AHLs (e.g., N-3-hydroxy-7-cis-tetradecenoyl-HSL) via accurate mass and fragmentation patterns .
- Bioinformatics : Genome mining identifies cryptic AHL synthases (e.g., hdtS in P. fluorescens) linked to uncharacterized QS systems .
Propiedades
IUPAC Name |
N-[(3S)-2-oxooxolan-3-yl]-2-phenylacetamide | |
---|---|---|
Source | PubChem | |
URL | https://pubchem.ncbi.nlm.nih.gov | |
Description | Data deposited in or computed by PubChem | |
InChI |
InChI=1S/C12H13NO3/c14-11(8-9-4-2-1-3-5-9)13-10-6-7-16-12(10)15/h1-5,10H,6-8H2,(H,13,14)/t10-/m0/s1 | |
Source | PubChem | |
URL | https://pubchem.ncbi.nlm.nih.gov | |
Description | Data deposited in or computed by PubChem | |
InChI Key |
YJTHUPUWKQRCLF-JTQLQIEISA-N | |
Source | PubChem | |
URL | https://pubchem.ncbi.nlm.nih.gov | |
Description | Data deposited in or computed by PubChem | |
Canonical SMILES |
C1COC(=O)C1NC(=O)CC2=CC=CC=C2 | |
Source | PubChem | |
URL | https://pubchem.ncbi.nlm.nih.gov | |
Description | Data deposited in or computed by PubChem | |
Isomeric SMILES |
C1COC(=O)[C@H]1NC(=O)CC2=CC=CC=C2 | |
Source | PubChem | |
URL | https://pubchem.ncbi.nlm.nih.gov | |
Description | Data deposited in or computed by PubChem | |
Molecular Formula |
C12H13NO3 | |
Source | PubChem | |
URL | https://pubchem.ncbi.nlm.nih.gov | |
Description | Data deposited in or computed by PubChem | |
Molecular Weight |
219.24 g/mol | |
Source | PubChem | |
URL | https://pubchem.ncbi.nlm.nih.gov | |
Description | Data deposited in or computed by PubChem | |
Retrosynthesis Analysis
AI-Powered Synthesis Planning: Our tool employs the Template_relevance Pistachio, Template_relevance Bkms_metabolic, Template_relevance Pistachio_ringbreaker, Template_relevance Reaxys, Template_relevance Reaxys_biocatalysis model, leveraging a vast database of chemical reactions to predict feasible synthetic routes.
One-Step Synthesis Focus: Specifically designed for one-step synthesis, it provides concise and direct routes for your target compounds, streamlining the synthesis process.
Accurate Predictions: Utilizing the extensive PISTACHIO, BKMS_METABOLIC, PISTACHIO_RINGBREAKER, REAXYS, REAXYS_BIOCATALYSIS database, our tool offers high-accuracy predictions, reflecting the latest in chemical research and data.
Strategy Settings
Precursor scoring | Relevance Heuristic |
---|---|
Min. plausibility | 0.01 |
Model | Template_relevance |
Template Set | Pistachio/Bkms_metabolic/Pistachio_ringbreaker/Reaxys/Reaxys_biocatalysis |
Top-N result to add to graph | 6 |
Feasible Synthetic Routes
Descargo de responsabilidad e información sobre productos de investigación in vitro
Tenga en cuenta que todos los artículos e información de productos presentados en BenchChem están destinados únicamente con fines informativos. Los productos disponibles para la compra en BenchChem están diseñados específicamente para estudios in vitro, que se realizan fuera de organismos vivos. Los estudios in vitro, derivados del término latino "in vidrio", involucran experimentos realizados en entornos de laboratorio controlados utilizando células o tejidos. Es importante tener en cuenta que estos productos no se clasifican como medicamentos y no han recibido la aprobación de la FDA para la prevención, tratamiento o cura de ninguna condición médica, dolencia o enfermedad. Debemos enfatizar que cualquier forma de introducción corporal de estos productos en humanos o animales está estrictamente prohibida por ley. Es esencial adherirse a estas pautas para garantizar el cumplimiento de los estándares legales y éticos en la investigación y experimentación.