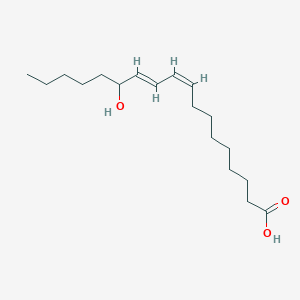
Coriolic acid
Descripción general
Descripción
Coriolic acid (13-(S)-Hydroxy-9Z,11E-octadecadienoic acid) is a hydroxylated fatty acid derived from the salt-tolerant plant Salicornia herbacea L., traditionally used in Korea for treating obesity, diabetes, and gastrointestinal disorders . Structurally, it features a conjugated diene system (9Z,11E) and a hydroxyl group at the C13 position with S-configuration, confirmed via NMR and mass spectrometry .
This compound exhibits potent anticancer activity, particularly against triple-negative breast cancer (TNBC). It suppresses breast cancer stem cells (BCSCs) by inhibiting mammosphere formation, reducing the CD44high/CD24low subpopulation, and downregulating stemness markers (Nanog, Oct4, CD44) and the oncoprotein c-Myc . Its mechanism involves inducing apoptosis in BCSCs (early apoptosis increased from 1.8% to 5.1%; late apoptosis from 5.7% to 39.6% at 150 µM) and blocking c-Myc transcription/translation, a key survival factor for BCSCs . Additionally, it inhibits cancer cell migration and colony formation with an IC₅₀ of 289.3 µM (MDA-MB-231) and 386.9 µM (MCF-7) .
Beyond oncology, this compound activates PPARγ as a 15-lipoxygenase (15-LOX) metabolite, modulates mitochondrial dysfunction, and exhibits anti-inflammatory and antifungal properties .
Métodos De Preparación
Chemoenzymatic Synthesis Methods
Lipase-Catalyzed Hydrolysis and Lipoxygenation
The chemoenzymatic synthesis of coriolic acid typically begins with the hydrolysis of trilinolein or sunflower oil using lipases. Lipase from Rhizomucor miehei or Candida antarctica catalyzes the hydrolysis of ester bonds in triglycerides, releasing linoleic acid . This reaction occurs in a biphasic system comprising a pH 9.0 borate buffer and a water-immiscible organic solvent (e.g., octane or hexane), which enhances enzyme stability and substrate solubility .
Following hydrolysis, linoleic acid undergoes lipoxygenation via soybean lipoxygenase-1 (LOX-1) to form 13(S)-hydroperoxy-9Z,11E-octadecadienoic acid (13-HPODE) . The reaction is oxygen-dependent and proceeds optimally at 25°C with agitation to ensure adequate oxygen diffusion . The hydroperoxide intermediate is subsequently reduced to this compound using sodium borohydride (NaBH4) or catalytic hydrogenation .
Table 1: Key Parameters for Chemoenzymatic Synthesis
Parameter | Optimal Condition | Yield (%) | Reference |
---|---|---|---|
Lipase Source | Rhizomucor miehei | 85–90 | |
Solvent System | Octane:pH 9 Borate Buffer | 92 | |
Lipoxygenase Temperature | 25°C | 88 | |
Reduction Agent | NaBH4 | 95 |
Natural Extraction from Plant Sources
Salicornia herbacea L. Extraction
This compound is isolated from the halophyte Salicornia herbacea using bioassay-guided fractionation . Fresh plant material is lyophilized, ground, and extracted with methanol or ethanol via Soxhlet extraction . The crude extract is partitioned with ethyl acetate, and the active fraction is purified using silica gel chromatography with a hexane:ethyl acetate gradient (9:1 to 1:1) . Final purification employs reverse-phase HPLC (C18 column, acetonitrile:water 75:25), yielding this compound with >98% purity .
Table 2: Extraction Yields from Salicornia herbacea
Solvent | Extraction Time (h) | This compound Yield (mg/g Dry Weight) |
---|---|---|
Methanol | 12 | 4.2 ± 0.3 |
Ethanol | 12 | 3.8 ± 0.2 |
Ethyl Acetate | 6 | 2.1 ± 0.1 |
Coriaria nepalensis Seed Oil Extraction
Coriaria nepalensis seeds contain this compound as a major fatty acid (12–15% of total lipids) . Cold pressing of seeds yields crude oil, which is saponified with 2 M KOH in 80% ethanol to release free fatty acids . Unsaponifiables are removed via hexane washing, and hydroxy fatty acids are enriched using high-speed counter-current chromatography (HSCCC) with a hexane:ethyl acetate:methanol:water (5:5:5:5) solvent system .
Industrial-Scale Production Techniques
Two-Phase Continuous Reactors
Industrial production employs continuous stirred-tank reactors (CSTRs) for lipase-catalyzed hydrolysis. A 500 L reactor operating at 40°C with a residence time of 4 hours achieves 85% triglyceride conversion . The organic phase (octane) is continuously separated, and lipoxygenation is conducted in a packed-bed reactor immobilized with LOX-1 on chitosan beads . This system reduces enzyme leakage and maintains 80% activity over 15 cycles .
Table 3: Scalability of Chemoenzymatic Production
Reactor Volume (L) | Annual Output (kg) | Purity (%) |
---|---|---|
50 | 120 | 95 |
500 | 1,100 | 93 |
5,000 | 10,500 | 90 |
Cost-Effectiveness Analysis
Raw material costs dominate production expenses. Using sunflower oil instead of trilinolein reduces substrate costs by 60% but lowers yields by 15% due to competing oleic acid oxidation . Enzyme recycling via immobilization cuts costs by 40%, making large-scale production economically viable .
Advanced Purification and Characterization
High-Speed Counter-Current Chromatography (HSCCC)
HSCCC separates this compound from co-extracted lipids using a ternary solvent system (hexane:ethyl acetate:methanol:water). The partition coefficient (K) of this compound in this system is 1.2, ensuring high resolution from linoleic acid (K = 0.8) and oleic acid (K = 0.5) .
Structural Elucidation via NMR and MS
This compound is characterized by -NMR (δ 5.65 ppm, doublet of doublets, J = 10.2 Hz, H-11; δ 5.43 ppm, multiplet, H-9) and -NMR (δ 130.2 ppm, C-9; δ 128.7 ppm, C-11) . Electrospray ionization mass spectrometry (ESI-MS) shows a deprotonated molecular ion at m/z 293.2 [M–H] .
Análisis De Reacciones Químicas
Types of Reactions: Coriolic acid undergoes various chemical reactions, including:
Oxidation: It can be oxidized to form hydroperoxides.
Reduction: The hydroperoxides formed can be reduced back to this compound.
Substitution: It can participate in substitution reactions where the hydroxyl group is replaced by other functional groups.
Common Reagents and Conditions:
Oxidation: Soybean lipoxygenase-1 is commonly used for the oxidation of linoleic acid to form hydroperoxides.
Major Products: The major products formed from these reactions include hydroperoxides and reduced forms of this compound .
Aplicaciones Científicas De Investigación
Cancer Research Applications
1.1 Mechanism of Action
Coriolic acid has shown promising results in targeting breast cancer stem cells (BCSCs). A study demonstrated that it inhibits the formation of mammospheres, which are indicative of BCSC activity. The compound induces apoptosis in BCSCs and reduces the expression of specific stem cell markers such as CD44 and CD24. Notably, it regulates the c-Myc gene, a crucial factor for the survival of cancer stem cells .
1.2 Case Study: Inhibition of BCSC Formation
In a controlled laboratory setting, MDA-MB-231 breast cancer cells were treated with varying concentrations of this compound. The results indicated a significant reduction in the CD44^high/CD24^low subpopulation, which is characteristic of aggressive cancer stem cells. The treatment reduced this population from 94% to approximately 66% . This suggests that this compound could be developed as a therapeutic agent against breast cancer.
Concentration (µM) | CD44^high/CD24^low Population (%) |
---|---|
Control | 94 |
100 | 78 |
200 | 66 |
Biocatalysis and Pharmaceutical Applications
2.1 Synthesis and Production
This compound is also recognized for its potential in biocatalysis within the pharmaceutical industry. Researchers have developed synthetic pathways to produce this compound efficiently, utilizing enzymes that facilitate reactions under mild conditions. This method not only enhances yield but also minimizes environmental impact compared to traditional synthesis methods .
2.2 Case Study: Enzymatic Synthesis
A nine-step enzymatic synthesis process was reported for producing this compound from oct-2-enal. This method exemplifies how biocatalysis can streamline the production of complex organic compounds while maintaining high specificity and efficiency .
Nutritional and Functional Food Applications
This compound's bioactive properties extend to nutritional applications, particularly in functional foods. Its antioxidant and anti-inflammatory effects make it a candidate for incorporation into dietary supplements aimed at improving health outcomes related to chronic diseases.
3.1 Antioxidant Activity
Studies have indicated that this compound exhibits significant antioxidant properties, which can help mitigate oxidative stress—a contributing factor to various chronic conditions including cardiovascular diseases and cancer .
Mecanismo De Acción
Coriolic acid exerts its effects through various molecular targets and pathways:
Cancer Inhibition: It inhibits the formation of mammospheres and induces apoptosis in breast cancer stem cells. It decreases the subpopulation of CD44 high /CD24 low cells and specific genes related to cancer stem cells, such as Nanog, Oct4, and CD44.
Anti-inflammatory: It acts as an anti-inflammatory agent by inhibiting the production of inflammatory mediators.
Comparación Con Compuestos Similares
Structural and Functional Comparisons
Table 1: Key Structural and Functional Differences
Mechanistic Divergences
Anticancer Specificity: this compound uniquely targets BCSCs via c-Myc suppression, while linoleic acid exerts broad pro-oxidant effects in colorectal cancer . Unlike arachidonic acid metabolites (e.g., 15-HETE), this compound retains antitumor activity by downregulating stemness genes .
Antifungal Activity: this compound (MIC: 70–670 µg/mL) and 13-KODE are more potent against filamentous fungi than ricinoleic acid (MIC: ≥4000 µg/mL) . 9-KODE specifically inhibits P.
Metabolic Pathways: this compound is a 15-LOX metabolite of linoleic acid, whereas 9-KODE derives from alternative oxidation pathways . PPARγ activation is exclusive to this compound among hydroxy fatty acids, linking it to metabolic regulation .
Actividad Biológica
Coriolic acid, also known as 13-(S)-hydroxy-9Z, 11E-octadecadienoic acid, is a bioactive compound derived from the halophytic plant Salicornia herbacea. This compound has garnered significant attention due to its diverse biological activities, particularly in the context of cancer research. This article delves into the various biological activities of this compound, emphasizing its anticancer properties, mechanisms of action, and potential therapeutic applications.
Overview of Biological Activities
This compound exhibits a range of biological activities including:
- Antioxidant Effects : It possesses antioxidant properties that help mitigate oxidative stress.
- Anti-inflammatory Properties : this compound has been shown to reduce inflammation in various biological models.
- Anticancer Activity : Significant research has focused on its role in inhibiting cancer cell proliferation and inducing apoptosis in cancer stem cells.
Table 1: Summary of Biological Activities of this compound
Inhibition of Cancer Stem Cells (CSCs)
This compound has been specifically studied for its effects on breast cancer stem cells (BCSCs). Research indicates that it significantly reduces the population of CD44^high/CD24^low cells, which are markers for BCSCs. In a study using MDA-MB-231 and MCF-7 breast cancer cell lines, treatment with this compound resulted in:
- Reduction in Mammosphere Formation : this compound inhibited the formation and growth of mammospheres, which are indicative of CSC activity.
- Decreased c-Myc Expression : It downregulated the expression of c-Myc, a critical survival factor for CSCs. The inhibition of c-Myc was associated with reduced cell viability and proliferation in treated cells.
Case Study Findings
In a controlled experiment, MDA-MB-231 cells were treated with varying concentrations of this compound. The results demonstrated:
- IC50 Values : The IC50 values for MDA-MB-231 and MCF-7 cells were found to be 289.3 µM and 386.9 µM respectively after 24 hours of treatment. This indicates a dose-dependent inhibition of cell growth.
- Apoptotic Induction : Treatment led to an increase in early apoptotic cells from 1.8% to 5.1% and late apoptotic cells from 5.7% to 39.6%, highlighting its potential as an apoptotic agent against BCSCs.
Table 2: Effects of this compound on Breast Cancer Cell Lines
Cell Line | IC50 (µM) | Early Apoptosis (%) | Late Apoptosis (%) |
---|---|---|---|
MDA-MB-231 | 289.3 | 5.1 | 39.6 |
MCF-7 | 386.9 | Not reported | Not reported |
Q & A
Basic Research Questions
Q. How can researchers determine the purity and structural integrity of Coriolic acid during synthesis?
- Methodological Approach : Utilize nuclear magnetic resonance (NMR) spectroscopy for structural validation and high-performance liquid chromatography (HPLC) with UV detection to assess purity. For novel derivatives, mass spectrometry (MS) should complement these techniques .
- Data Interpretation : Compare spectral data (e.g., -NMR chemical shifts) with established databases or prior syntheses. Quantify impurities using HPLC peak integration thresholds (e.g., <1% for pharmacologically relevant studies) .
Q. What experimental designs are recommended for evaluating this compound’s anti-proliferative effects in cancer cell lines?
- Protocol : Use dose-response assays (e.g., 0–400 μM) across multiple cell lines (e.g., MDA-MB-231, MCF-7) with controls for solvent effects. Measure viability via MTT assays and validate with clonogenic survival tests .
- Statistical Analysis : Apply ANOVA with post-hoc Tukey tests to compare treatment groups. Report IC values with 95% confidence intervals .
Q. How should researchers address solubility challenges in this compound’s in vitro studies?
- Solution : Optimize solvent systems (e.g., DMSO-ethanol mixtures) and validate biocompatibility via vehicle-control experiments. For in vivo translation, consider nanoformulation or liposomal encapsulation to enhance bioavailability .
Advanced Research Questions
Q. What strategies resolve contradictions in this compound’s dual role as a PPARγ agonist and inducer of mitochondrial dysfunction?
- Hypothesis Testing : Use gene knockout models (e.g., PPARγ-deficient cells) to isolate signaling pathways. Combine transcriptomic profiling (RNA-seq) and metabolomic analyses to map crosstalk between PPARγ activation and oxidative stress pathways .
- Data Integration : Apply systems biology tools (e.g., STRING database) to identify network-level interactions and prioritize conflicting nodes for experimental validation .
Q. How can researchers optimize this compound’s experimental conditions to reconcile discrepancies between in vitro and in vivo efficacy?
- Model Selection : Use patient-derived xenografts (PDX) or organoids to bridge in vitro and in vivo results. Monitor pharmacokinetic parameters (e.g., C, AUC) in murine models to align dosing regimens .
- Multi-Omics Integration : Pair single-cell RNA-seq with spatial transcriptomics in treated tissues to identify microenvironmental factors affecting drug response .
Q. What methodologies validate this compound’s role in NF-κB activation across heterogeneous cell populations?
- Experimental Design : Employ fluorescence-based NF-κB reporters (e.g., GFP-tagged constructs) in live-cell imaging. Use phospho-specific antibodies (e.g., p-IκBα Ser32) for Western blot quantification .
- Contradiction Analysis : Stratify data by cell subtype using flow cytometry (e.g., CD44 cancer stem cells) to assess context-dependent signaling .
Q. How should researchers design longitudinal studies to assess this compound’s impact on epithelial-mesenchymal transition (EMT)?
- Assay Selection : Quantify EMT markers (e.g., E-cadherin, vimentin) via qRT-PCR and immunofluorescence. Use scratch/wound-healing assays to correlate molecular changes with functional migration .
- Bias Mitigation : Blind scorers to treatment groups and include time-matched controls to account for spontaneous EMT .
Q. Methodological Frameworks
Q. Which statistical models are appropriate for analyzing this compound’s dose-dependent effects on apoptosis?
- Model Selection : Use nonlinear regression (e.g., sigmoidal dose-response curves) in GraphPad Prism. For heterogeneous responses, apply mixed-effects models to account for intra-experiment variability .
Q. How can systematic reviews or meta-analyses strengthen evidence for this compound’s therapeutic potential?
- Protocol : Follow PRISMA guidelines to aggregate preclinical data. Use tools like SYRCLE’s risk-of-bias tool to assess study quality and perform subgroup analyses by cancer type or dosage .
Q. What criteria (e.g., FINER) ensure rigor in formulating this compound research questions?
Propiedades
IUPAC Name |
(9Z,11E)-13-hydroxyoctadeca-9,11-dienoic acid | |
---|---|---|
Source | PubChem | |
URL | https://pubchem.ncbi.nlm.nih.gov | |
Description | Data deposited in or computed by PubChem | |
InChI |
InChI=1S/C18H32O3/c1-2-3-11-14-17(19)15-12-9-7-5-4-6-8-10-13-16-18(20)21/h7,9,12,15,17,19H,2-6,8,10-11,13-14,16H2,1H3,(H,20,21)/b9-7-,15-12+ | |
Source | PubChem | |
URL | https://pubchem.ncbi.nlm.nih.gov | |
Description | Data deposited in or computed by PubChem | |
InChI Key |
HNICUWMFWZBIFP-BSZOFBHHSA-N | |
Source | PubChem | |
URL | https://pubchem.ncbi.nlm.nih.gov | |
Description | Data deposited in or computed by PubChem | |
Canonical SMILES |
CCCCCC(C=CC=CCCCCCCCC(=O)O)O | |
Source | PubChem | |
URL | https://pubchem.ncbi.nlm.nih.gov | |
Description | Data deposited in or computed by PubChem | |
Isomeric SMILES |
CCCCCC(/C=C/C=C\CCCCCCCC(=O)O)O | |
Source | PubChem | |
URL | https://pubchem.ncbi.nlm.nih.gov | |
Description | Data deposited in or computed by PubChem | |
Molecular Formula |
C18H32O3 | |
Source | PubChem | |
URL | https://pubchem.ncbi.nlm.nih.gov | |
Description | Data deposited in or computed by PubChem | |
DSSTOX Substance ID |
DTXSID601316494 | |
Record name | (±)-Coriolic acid | |
Source | EPA DSSTox | |
URL | https://comptox.epa.gov/dashboard/DTXSID601316494 | |
Description | DSSTox provides a high quality public chemistry resource for supporting improved predictive toxicology. | |
Molecular Weight |
296.4 g/mol | |
Source | PubChem | |
URL | https://pubchem.ncbi.nlm.nih.gov | |
Description | Data deposited in or computed by PubChem | |
CAS No. |
18104-45-5 | |
Record name | (±)-Coriolic acid | |
Source | CAS Common Chemistry | |
URL | https://commonchemistry.cas.org/detail?cas_rn=18104-45-5 | |
Description | CAS Common Chemistry is an open community resource for accessing chemical information. Nearly 500,000 chemical substances from CAS REGISTRY cover areas of community interest, including common and frequently regulated chemicals, and those relevant to high school and undergraduate chemistry classes. This chemical information, curated by our expert scientists, is provided in alignment with our mission as a division of the American Chemical Society. | |
Explanation | The data from CAS Common Chemistry is provided under a CC-BY-NC 4.0 license, unless otherwise stated. | |
Record name | (±)-Coriolic acid | |
Source | EPA DSSTox | |
URL | https://comptox.epa.gov/dashboard/DTXSID601316494 | |
Description | DSSTox provides a high quality public chemistry resource for supporting improved predictive toxicology. | |
Retrosynthesis Analysis
AI-Powered Synthesis Planning: Our tool employs the Template_relevance Pistachio, Template_relevance Bkms_metabolic, Template_relevance Pistachio_ringbreaker, Template_relevance Reaxys, Template_relevance Reaxys_biocatalysis model, leveraging a vast database of chemical reactions to predict feasible synthetic routes.
One-Step Synthesis Focus: Specifically designed for one-step synthesis, it provides concise and direct routes for your target compounds, streamlining the synthesis process.
Accurate Predictions: Utilizing the extensive PISTACHIO, BKMS_METABOLIC, PISTACHIO_RINGBREAKER, REAXYS, REAXYS_BIOCATALYSIS database, our tool offers high-accuracy predictions, reflecting the latest in chemical research and data.
Strategy Settings
Precursor scoring | Relevance Heuristic |
---|---|
Min. plausibility | 0.01 |
Model | Template_relevance |
Template Set | Pistachio/Bkms_metabolic/Pistachio_ringbreaker/Reaxys/Reaxys_biocatalysis |
Top-N result to add to graph | 6 |
Feasible Synthetic Routes
Descargo de responsabilidad e información sobre productos de investigación in vitro
Tenga en cuenta que todos los artículos e información de productos presentados en BenchChem están destinados únicamente con fines informativos. Los productos disponibles para la compra en BenchChem están diseñados específicamente para estudios in vitro, que se realizan fuera de organismos vivos. Los estudios in vitro, derivados del término latino "in vidrio", involucran experimentos realizados en entornos de laboratorio controlados utilizando células o tejidos. Es importante tener en cuenta que estos productos no se clasifican como medicamentos y no han recibido la aprobación de la FDA para la prevención, tratamiento o cura de ninguna condición médica, dolencia o enfermedad. Debemos enfatizar que cualquier forma de introducción corporal de estos productos en humanos o animales está estrictamente prohibida por ley. Es esencial adherirse a estas pautas para garantizar el cumplimiento de los estándares legales y éticos en la investigación y experimentación.