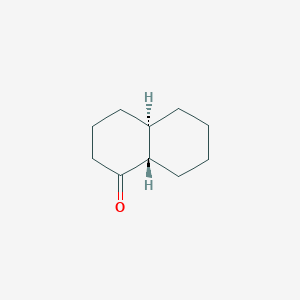
trans-1-Decalone
Descripción general
Descripción
Trans-1-Decalone is a synthetic cyclopentanone derivative . It is a member of the ketone class of compounds and holds significant potential in laboratory experiments and scientific research . It has been found to have antimicrobial and antioxidant activity of essential oils isolated from Citrus aurantium .
Synthesis Analysis
The synthesis of trans-1-Decalone involves the Fischer indolisation of trans-1-decalone . The regioselectivity of this process has been examined to define conditions which select either indole or indolenine products . During the reactions, aerial oxidation may occur, leading to some unexpected rearrangements .Molecular Structure Analysis
Trans-1-Decalone is a cyclopentanone derivative . The molecular formula is C10H16O . The structure of trans-1-Decalone can be viewed using Java or Javascript .Chemical Reactions Analysis
The chemical reactions of trans-1-Decalone involve the Fischer indolisation . During the reactions, aerial oxidation may occur, leading to some unexpected rearrangements . The reactions of the indolenines with nucleophiles have also been studied .Physical And Chemical Properties Analysis
Trans-1-Decalone has a molecular weight of 152.23 g/mol . It has a boiling point of 73 °C/1 mmHg (lit.) and a melting point of 30-32 °C (lit.) .Aplicaciones Científicas De Investigación
Anti-Inflammatory Applications
trans-1-Decalone: has been identified as a potential anti-inflammatory agent. It is believed to inhibit the production of pro-inflammatory cytokines by acting as an inhibitor of the enzyme cyclooxygenase-2 (COX-2) . This property could be harnessed in the development of new anti-inflammatory drugs, particularly for conditions where COX-2 is implicated, such as arthritis and other inflammatory diseases.
Anti-Tumor Agent
Research has explored trans-1-Decalone for its effectiveness as an anti-tumor agent. It may impede cancer cell proliferation, offering a new avenue for cancer treatment. The compound’s ability to inhibit key enzymes involved in cell growth could be pivotal in developing novel anti-cancer therapies .
Neurological Disorder Treatment
In the realm of neurology, trans-1-Decalone shows promise as a treatment for neurological disorders by affecting dopamine levels in the brain. Its modulation of acetylcholinesterase, an enzyme responsible for breaking down the neurotransmitter acetylcholine, suggests potential applications in treating conditions like Alzheimer’s disease .
Antibacterial Activities
trans-Bicyclo(4.4.0)decan-1-one: derivatives are noted for their potent and selective antibacterial activities. They are part of a class of compounds that have been used in the development of new antibiotics, particularly in the fight against antibiotic-resistant bacteria . The structural diversity of these compounds allows for the targeting of a wide range of bacterial pathogens.
Synthetic Chemistry
In synthetic chemistry, trans-1-Decalone serves as a building block for more complex chemical structures. Its incorporation into multifunctional frameworks is crucial for synthesizing natural products, especially those with antibiotic properties . The compound’s versatility makes it a valuable asset in the chemist’s toolkit for creating diverse molecular architectures.
Enzyme Inhibition Studies
The compound’s ability to inhibit various enzymes, such as 5-lipoxygenase (5-LOX) , which is involved in the inflammatory response, makes it a useful tool in biochemical studies. Understanding how trans-1-Decalone interacts with these enzymes can lead to the discovery of new therapeutic targets and the development of drugs to treat inflammatory conditions .
Optical Rotatory Dispersion Studies
trans-1-Decalone: has been used in optical rotatory dispersion studies to understand the conformational properties of molecules. These studies are essential for determining the three-dimensional arrangement of atoms in a molecule, which is crucial for predicting its reactivity and interaction with biological systems .
Proteomics Research
Lastly, trans-1-Decalone is utilized in proteomics research. It can be used as a substrate for various enzymatic reactions, aiding in the study of protein functions and interactions. This application is vital for understanding the complex biological processes and developing targeted therapies for diseases .
Mecanismo De Acción
Target of Action
Trans-1-Decalone, also known as trans-Bicyclo(4.4.0)decan-1-one, is a synthetic cyclopentanone derivative . It primarily targets the enzyme cyclooxygenase-2 (COX-2) , which is responsible for producing pro-inflammatory cytokines . It also inhibits the activity of the enzyme 5-lipoxygenase (5-LOX) , which contributes to the production of leukotrienes involved in the inflammatory response . Furthermore, it modulates the activity of acetylcholinesterase , an enzyme responsible for acetylcholine breakdown, thus affecting neurotransmitter levels .
Mode of Action
Trans-1-Decalone interacts with its targets by functioning as an inhibitor. It inhibits COX-2, thereby reducing the production of pro-inflammatory cytokines . It also demonstrates the ability to inhibit 5-LOX, reducing the production of leukotrienes . By modulating the activity of acetylcholinesterase, it affects the levels of the neurotransmitter acetylcholine .
Biochemical Pathways
The biochemical pathways affected by trans-1-Decalone are primarily those involved in inflammation and neurotransmission. By inhibiting COX-2 and 5-LOX, it impacts the inflammatory response pathway . The modulation of acetylcholinesterase activity affects the cholinergic neurotransmission pathway .
Pharmacokinetics
As a member of the ketone class of compounds, it may share similar pharmacokinetic properties with other ketones .
Result of Action
The action of trans-1-Decalone results in several molecular and cellular effects. It has been explored for its potential as an anti-inflammatory agent due to its ability to inhibit the production of pro-inflammatory cytokines . Its effectiveness as an anti-tumor agent has also been investigated due to its ability to impede cancer cell proliferation . Furthermore, trans-1-Decalone has shown promise as a potential treatment for neurological disorders by reducing dopamine levels in the brain .
Action Environment
Like other chemical compounds, its action and stability could potentially be influenced by factors such as temperature, ph, and the presence of other compounds .
Direcciones Futuras
Trans-1-Decalone holds significant potential in laboratory experiments and scientific research . It has been explored for its potential as an anti-inflammatory agent by inhibiting the production of pro-inflammatory cytokines . Its effectiveness as an anti-tumor agent has also been investigated due to its ability to impede cancer cell proliferation . Furthermore, it has shown promise as a potential treatment for neurological disorders by reducing dopamine levels in the brain .
Propiedades
IUPAC Name |
(4aR,8aS)-3,4,4a,5,6,7,8,8a-octahydro-2H-naphthalen-1-one | |
---|---|---|
Source | PubChem | |
URL | https://pubchem.ncbi.nlm.nih.gov | |
Description | Data deposited in or computed by PubChem | |
InChI |
InChI=1S/C10H16O/c11-10-7-3-5-8-4-1-2-6-9(8)10/h8-9H,1-7H2/t8-,9+/m1/s1 | |
Source | PubChem | |
URL | https://pubchem.ncbi.nlm.nih.gov | |
Description | Data deposited in or computed by PubChem | |
InChI Key |
AFEFRXAPJRCTOW-BDAKNGLRSA-N | |
Source | PubChem | |
URL | https://pubchem.ncbi.nlm.nih.gov | |
Description | Data deposited in or computed by PubChem | |
Canonical SMILES |
C1CCC2C(C1)CCCC2=O | |
Source | PubChem | |
URL | https://pubchem.ncbi.nlm.nih.gov | |
Description | Data deposited in or computed by PubChem | |
Isomeric SMILES |
C1CC[C@H]2[C@H](C1)CCCC2=O | |
Source | PubChem | |
URL | https://pubchem.ncbi.nlm.nih.gov | |
Description | Data deposited in or computed by PubChem | |
Molecular Formula |
C10H16O | |
Source | PubChem | |
URL | https://pubchem.ncbi.nlm.nih.gov | |
Description | Data deposited in or computed by PubChem | |
DSSTOX Substance ID |
DTXSID301030742 | |
Record name | trans-Bicyclo(4.4.0)decan-1-one | |
Source | EPA DSSTox | |
URL | https://comptox.epa.gov/dashboard/DTXSID301030742 | |
Description | DSSTox provides a high quality public chemistry resource for supporting improved predictive toxicology. | |
Molecular Weight |
152.23 g/mol | |
Source | PubChem | |
URL | https://pubchem.ncbi.nlm.nih.gov | |
Description | Data deposited in or computed by PubChem | |
Product Name |
trans-1-Decalone | |
CAS RN |
936-18-5, 21370-71-8 | |
Record name | (4aR,8aS)-Octahydro-1(2H)-naphthalenone | |
Source | CAS Common Chemistry | |
URL | https://commonchemistry.cas.org/detail?cas_rn=936-18-5 | |
Description | CAS Common Chemistry is an open community resource for accessing chemical information. Nearly 500,000 chemical substances from CAS REGISTRY cover areas of community interest, including common and frequently regulated chemicals, and those relevant to high school and undergraduate chemistry classes. This chemical information, curated by our expert scientists, is provided in alignment with our mission as a division of the American Chemical Society. | |
Explanation | The data from CAS Common Chemistry is provided under a CC-BY-NC 4.0 license, unless otherwise stated. | |
Record name | rel-(4aR,8aS)-Octahydro-1(2H)-naphthalenone | |
Source | CAS Common Chemistry | |
URL | https://commonchemistry.cas.org/detail?cas_rn=21370-71-8 | |
Description | CAS Common Chemistry is an open community resource for accessing chemical information. Nearly 500,000 chemical substances from CAS REGISTRY cover areas of community interest, including common and frequently regulated chemicals, and those relevant to high school and undergraduate chemistry classes. This chemical information, curated by our expert scientists, is provided in alignment with our mission as a division of the American Chemical Society. | |
Explanation | The data from CAS Common Chemistry is provided under a CC-BY-NC 4.0 license, unless otherwise stated. | |
Record name | trans-1-Decalone | |
Source | ChemIDplus | |
URL | https://pubchem.ncbi.nlm.nih.gov/substance/?source=chemidplus&sourceid=0021370718 | |
Description | ChemIDplus is a free, web search system that provides access to the structure and nomenclature authority files used for the identification of chemical substances cited in National Library of Medicine (NLM) databases, including the TOXNET system. | |
Record name | trans-Bicyclo(4.4.0)decan-1-one | |
Source | EPA DSSTox | |
URL | https://comptox.epa.gov/dashboard/DTXSID301030742 | |
Description | DSSTox provides a high quality public chemistry resource for supporting improved predictive toxicology. | |
Record name | trans-bicyclo(4.4.0)decan-1-one | |
Source | European Chemicals Agency (ECHA) | |
URL | https://echa.europa.eu/substance-information/-/substanceinfo/100.040.305 | |
Description | The European Chemicals Agency (ECHA) is an agency of the European Union which is the driving force among regulatory authorities in implementing the EU's groundbreaking chemicals legislation for the benefit of human health and the environment as well as for innovation and competitiveness. | |
Explanation | Use of the information, documents and data from the ECHA website is subject to the terms and conditions of this Legal Notice, and subject to other binding limitations provided for under applicable law, the information, documents and data made available on the ECHA website may be reproduced, distributed and/or used, totally or in part, for non-commercial purposes provided that ECHA is acknowledged as the source: "Source: European Chemicals Agency, http://echa.europa.eu/". Such acknowledgement must be included in each copy of the material. ECHA permits and encourages organisations and individuals to create links to the ECHA website under the following cumulative conditions: Links can only be made to webpages that provide a link to the Legal Notice page. | |
Record name | TRANS-1-DECALONE | |
Source | FDA Global Substance Registration System (GSRS) | |
URL | https://gsrs.ncats.nih.gov/ginas/app/beta/substances/22P3A7D875 | |
Description | The FDA Global Substance Registration System (GSRS) enables the efficient and accurate exchange of information on what substances are in regulated products. Instead of relying on names, which vary across regulatory domains, countries, and regions, the GSRS knowledge base makes it possible for substances to be defined by standardized, scientific descriptions. | |
Explanation | Unless otherwise noted, the contents of the FDA website (www.fda.gov), both text and graphics, are not copyrighted. They are in the public domain and may be republished, reprinted and otherwise used freely by anyone without the need to obtain permission from FDA. Credit to the U.S. Food and Drug Administration as the source is appreciated but not required. | |
Retrosynthesis Analysis
AI-Powered Synthesis Planning: Our tool employs the Template_relevance Pistachio, Template_relevance Bkms_metabolic, Template_relevance Pistachio_ringbreaker, Template_relevance Reaxys, Template_relevance Reaxys_biocatalysis model, leveraging a vast database of chemical reactions to predict feasible synthetic routes.
One-Step Synthesis Focus: Specifically designed for one-step synthesis, it provides concise and direct routes for your target compounds, streamlining the synthesis process.
Accurate Predictions: Utilizing the extensive PISTACHIO, BKMS_METABOLIC, PISTACHIO_RINGBREAKER, REAXYS, REAXYS_BIOCATALYSIS database, our tool offers high-accuracy predictions, reflecting the latest in chemical research and data.
Strategy Settings
Precursor scoring | Relevance Heuristic |
---|---|
Min. plausibility | 0.01 |
Model | Template_relevance |
Template Set | Pistachio/Bkms_metabolic/Pistachio_ringbreaker/Reaxys/Reaxys_biocatalysis |
Top-N result to add to graph | 6 |
Feasible Synthetic Routes
Q & A
Q1: What is the molecular formula and weight of trans-1-Decalone?
A1: Trans-1-Decalone has the molecular formula C10H16O and a molecular weight of 152.23 g/mol.
Q2: How can one differentiate between cis and trans-1-decalone using spectroscopic techniques?
A2: Carbon-13 Nuclear Magnetic Resonance (13C NMR) spectroscopy provides a reliable method for differentiating between cis and trans-1-decalone isomers. []
Q3: Can trans-1-Decalone be synthesized asymmetrically?
A3: Yes, a key reagent for achieving highly enantioselective synthesis of trans-1-Decalone is Isopinocampheylchloroborane. This reagent enables the conversion of 1-Allyl-1-cyclohexene into trans-1-Decalone with an optical purity greater than or equal to 99%. [, ]
Q4: Can trans-1-Decalone be used in the synthesis of complex molecules?
A4: Absolutely! Trans-1-Decalone serves as a versatile starting material for synthesizing various compounds, including 5,5,9-trimethyl-trans-1-decalone and 6β-hydroxy-5,5,9β-trimethyl-trans-1-decalone. [, ]
Q5: Are there any examples of stereoselective reactions involving trans-1-Decalone?
A5: Yes, the reaction of 9-Chloro-trans-1-decalone with methoxide ions exhibits stereoselectivity. [] Additionally, alkylation studies of the δ19-enolate of anti-6-t-butyl-cis and trans-1-decalone demonstrate stereoselective behavior. []
Q6: How does trans-1-Decalone behave under electrochemical conditions?
A6: Electrochemical catalysis in microemulsions allows for the stereoselective production of trans-1-Decalone. This method provides control over the stereochemical outcome of the reaction. []
Q7: What happens when 2-Furfurylidene-10-methyl-trans-1-decalone is treated with a base?
A7: Treating 2-Furfurylidene-10-methyl-trans-1-decalone with a base (NaOH – aqueous HOCH2CH2OH) leads to the formation of nine distinct products. The structures of eight of these products have been successfully elucidated. [, ]
Q8: Can trans-1-Decalone be functionalized for further synthetic manipulations?
A8: Yes, the 2-isopropylthiomethylene derivatives of trans-1-Decalone react with lithium tetramethylpiperidide and methyl acrylate to yield cycloadducts. These adducts can then be transformed into annelated cyclopentenones, showcasing the potential for building complex structures from trans-1-Decalone. [, ]
Q9: Have computational methods been applied to study trans-1-Decalone and its derivatives?
A9: Yes, molecular mechanics (MM2) calculations have been used to understand the conformational preferences of macrocyclic α-keto radical intermediates derived from trans-1-Decalone derivatives. These calculations provide insights into the regioselectivity observed in radical cyclization reactions. []
Q10: Does trans-1-Decalone interact with biological systems?
A10: While trans-1-Decalone itself may not have direct biological targets, its structure closely resembles that of natural products. For instance, analogs of steroid compounds lacking the B ring have been synthesized, and trans-1-Decalone could potentially serve as a scaffold for such molecules. []
Q11: Are there any enzymes known to interact with trans-1-Decalone or its derivatives?
A11: Yes, studies on the metabolism of isomeric decalones in rabbits revealed that both cis and trans-2-Decalone are reduced to racemic secondary alcohols possessing an equatorial hydroxyl group. [] This suggests the involvement of reductase enzymes in the metabolism of decalones.
Q12: Can trans-1-Decalone be used to study enzyme activity and selectivity?
A12: Research has shown that trans-1-Decalone acts as a substrate for dihydroxyacetone reductase from the fungus Mucor javanicus. [] This enzyme exhibits enantioselectivity, preferentially reducing one enantiomer of trans-1-Decalone over the other. Further, it showcases stereospecificity with respect to the coenzyme, transferring a hydride from a specific face. These findings highlight the potential of trans-1-Decalone and its derivatives as tools for investigating enzyme stereoselectivity.
Q13: Have any studies explored the use of computational methods to understand the interaction of trans-1-Decalone with enzymes?
A13: Yes, atomistic simulations have been employed to investigate the stereoselectivity of Actinorhodin Ketoreductase (actKR) variants using trans-1-Decalone as a model substrate. [] Classical molecular dynamics (MD) simulations, binding free energy calculations using MM/PBSA, and hybrid QM/MM MD simulations with umbrella sampling, benchmarked with density functional theory, were employed. These studies provided insights into the factors governing the enzyme's preference for producing specific stereoisomers of trans-1-Decalol from trans-1-Decalone.
Descargo de responsabilidad e información sobre productos de investigación in vitro
Tenga en cuenta que todos los artículos e información de productos presentados en BenchChem están destinados únicamente con fines informativos. Los productos disponibles para la compra en BenchChem están diseñados específicamente para estudios in vitro, que se realizan fuera de organismos vivos. Los estudios in vitro, derivados del término latino "in vidrio", involucran experimentos realizados en entornos de laboratorio controlados utilizando células o tejidos. Es importante tener en cuenta que estos productos no se clasifican como medicamentos y no han recibido la aprobación de la FDA para la prevención, tratamiento o cura de ninguna condición médica, dolencia o enfermedad. Debemos enfatizar que cualquier forma de introducción corporal de estos productos en humanos o animales está estrictamente prohibida por ley. Es esencial adherirse a estas pautas para garantizar el cumplimiento de los estándares legales y éticos en la investigación y experimentación.