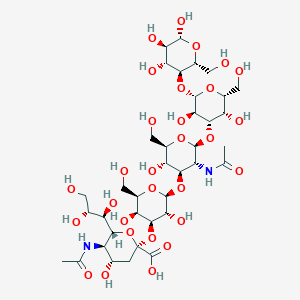
GM1-Pentasaccharide
Descripción general
Descripción
GM1-Pentasaccharide is a glycosphingolipid, specifically a ganglioside, which consists of a pentasaccharide chain attached to a ceramide moiety. It is a crucial component of the cell membrane in the central nervous system and plays a significant role in various neurological functions. This compound is known for its involvement in cell differentiation, neuritogenesis, neuroregeneration, and signal transduction .
Métodos De Preparación
Synthetic Routes and Reaction Conditions: The synthesis of GM1-Pentasaccharide typically involves a [3+2] strategy. The GM3 trisaccharide acceptor, bearing an azido propyl group at the reducing end, is prepared using traditional acetamide-protected sialyl thioglycosyl donors. The glycosylation of the axial 4-hydroxyl group of GM3 by the disaccharide donor is highly dependent on donor protective groups. The fully protected this compound is obtained by deprotection of the pentasaccharide .
Industrial Production Methods: Industrial production of this compound can be achieved through biosynthesis on lactose-immobilized surfaces using glycosyltransferase reactions. This method involves three consecutive glycosyltransferase reactions along with small amounts of enzymes and donors, without any additional processes .
Análisis De Reacciones Químicas
Types of Reactions: GM1-Pentasaccharide undergoes various chemical reactions, including glycosylation, deprotection, and enzymatic glycosylation.
Common Reagents and Conditions:
Glycosylation: Traditional acetamide-protected sialyl thioglycosyl donors are used.
Deprotection: Specific conditions for removing protective groups to yield the functionalized pentasaccharide.
Enzymatic Glycosylation: Utilizes glycosyltransferase enzymes for biosynthesis on immobilized surfaces.
Major Products: The major products formed from these reactions include the fully protected this compound and its functionalized derivatives .
Aplicaciones Científicas De Investigación
Neurological Applications
1.1 Gene Therapy for GM1 Gangliosidosis
GM1 gangliosidosis is a rare neurodegenerative disorder caused by mutations in the GLB1 gene, leading to β-galactosidase deficiency. Recent studies have identified H3N2b, a pentasaccharide biomarker related to GM1, as a significant indicator for monitoring the efficacy of gene therapy. In a study involving a cat model and human patients, H3N2b levels were found to be elevated in plasma, cerebrospinal fluid (CSF), and urine of affected individuals. Following adeno-associated viral (AAV) gene therapy, reductions in H3N2b levels correlated with improvements in clinical outcomes and neuropathology, suggesting its potential as a pharmacodynamic biomarker for therapeutic response in GM1 gangliosidosis .
Table 1: Summary of Findings on H3N2b Biomarker
Sample Type | Pre-Treatment H3N2b Level | Post-Treatment H3N2b Level | Clinical Outcome Improvement |
---|---|---|---|
Cat Model CNS | Elevated | Reduced | Improved neuropathology |
Patient Plasma | Elevated | Reduced | Improved clinical outcomes |
Patient CSF | Elevated | Reduced | Improved clinical outcomes |
Diagnostic Applications
2.1 Autoantibody Detection in Peripheral Neuropathies
Anti-GM1 autoantibodies are utilized as diagnostic markers for motor axonal peripheral neuropathies. These antibodies can bind to GM1-rich membranes and are implicated in the pathogenesis of these neuropathies. Research indicates that the presence of these autoantibodies can lead to morphological damage at nerve terminals, highlighting their role in diagnosing and understanding neuropathic conditions .
Table 2: Characteristics of Anti-GM1 Autoantibodies
Feature | Description |
---|---|
Target | GM1 ganglioside-rich membranes |
Implication | Diagnostic marker for neuropathies |
Pathogenic Effect | Morphological damage at nerve terminals |
Therapeutic Applications
3.1 Cholera Toxin Inhibition
GM1-pentasaccharide has been explored as a potential therapeutic agent against cholera toxin. Studies have demonstrated that GM1 mimics can effectively block the binding sites of cholera toxin, offering a promising avenue for developing low-cost prophylactic treatments. These compounds can serve as receptor decoys, potentially alleviating symptoms without resorting to antibiotics .
Table 3: Inhibition Mechanism of GM1 Mimics Against Cholera Toxin
Mechanism | Description |
---|---|
Binding Site Blockage | GM1 mimics inhibit cholera toxin binding |
Decoy Function | Mimics act as receptors to reduce toxicity |
Synthesis Advantage | Relatively easy synthesis; resistant to degradation |
Future Directions and Research Opportunities
The ongoing research into this compound suggests several promising avenues:
- Further Validation as a Biomarker: Continued studies are needed to establish H3N2b's reliability across larger patient cohorts and different clinical settings.
- Exploration in Other Neurological Disorders: Investigating the role of this compound in other neurodegenerative diseases could unveil broader therapeutic applications.
- Development of Multivalent Inhibitors: Enhancing the efficacy of cholera toxin inhibitors through multivalent approaches may lead to more effective treatments.
Mecanismo De Acción
The mechanism of action of GM1-Pentasaccharide involves its direct interaction and activation of the membrane tyrosine kinase receptor, TrkA, which serves as the nerve growth factor receptor. This interaction is mediated by the oligosaccharide portion of this compound, leading to neuronal differentiation and trophism .
Comparación Con Compuestos Similares
GM2 Tetrasaccharide: A precursor in the biosynthesis of GM1-Pentasaccharide.
GM3 Trisaccharide: Another precursor in the biosynthesis pathway.
Fucosyl-GM1 Oligosaccharide: Similar in structure but with an additional fucose residue
Uniqueness: this compound is unique due to its specific interaction with the TrkA receptor, which is not observed with other similar compounds. This unique interaction underlies its neurotrophic and neuroprotective properties .
Actividad Biológica
GM1-pentasaccharide, a derivative of the ganglioside GM1, has garnered attention due to its biological significance, particularly in the context of neurodegenerative diseases and therapeutic applications. This article provides a comprehensive overview of its biological activity, supported by recent research findings, data tables, and case studies.
Overview of this compound
GM1 ganglioside is a glycosphingolipid found in the nervous system, playing crucial roles in cell signaling and neuroprotection. The pentasaccharide form of GM1 consists of five sugar units and is implicated in various biological processes, including immune responses and neuronal function.
Biological Activity
1. Role as a Biomarker in GM1 Gangliosidosis
Recent studies have identified the H3N2b pentasaccharide as a significant biomarker for GM1 gangliosidosis, a rare neurodegenerative disorder caused by deficiencies in the enzyme β-galactosidase. Research utilizing liquid chromatography-tandem mass spectrometry (LC-MS/MS) revealed that H3N2b levels were elevated over 18-fold in patients' plasma, cerebrospinal fluid (CSF), and urine compared to healthy controls .
Table 1: Comparison of H3N2b Levels
Sample Type | Control (Mean) | GM1 Gangliosidosis (Mean) | Fold Change |
---|---|---|---|
Plasma | 0.5 ng/mL | 9.5 ng/mL | 19 |
Cerebrospinal Fluid | 0.3 ng/mL | 5.7 ng/mL | 19 |
Urine | 0.2 ng/mL | 4.0 ng/mL | 20 |
2. Therapeutic Potential in Gene Therapy
The reduction of H3N2b levels following adeno-associated viral (AAV) gene therapy treatment was correlated with improvements in clinical outcomes and neurological symptoms in both animal models and human patients . This demonstrates the potential of this compound not only as a biomarker but also as a therapeutic target.
3. Immunological Implications
Anti-GM1 antibodies have been implicated in autoimmune neuropathies such as Guillain-Barré syndrome. These antibodies can bind to GM1 gangliosides on neuronal membranes, leading to nerve injury . The pentasaccharide structure may influence the binding affinity and pathogenicity of these antibodies, suggesting that this compound could play a role in modulating immune responses.
Case Studies
Case Study 1: Gene Therapy Efficacy
In a study involving GM1 gangliosidosis cats treated with AAV9 gene therapy, researchers observed significant reductions in H3N2b levels post-treatment. This correlated with improved neurological function and extended lifespan . The findings suggest that monitoring H3N2b could serve as an effective pharmacodynamic marker for assessing therapeutic efficacy.
Case Study 2: Autoimmune Neuropathy
A cohort study examined patients with motor axonal peripheral neuropathies associated with anti-GM1 antibodies. The presence of these antibodies was linked to increased levels of inflammatory markers and neurodegeneration . Understanding the interactions between this compound and these autoantibodies could provide insights into novel therapeutic strategies.
Propiedades
IUPAC Name |
(2S,4S,5R,6R)-5-acetamido-2-[(2R,3R,4S,5S,6R)-2-[(2S,3R,4R,5S,6R)-3-acetamido-2-[(2R,3S,4S,5R,6S)-3,5-dihydroxy-2-(hydroxymethyl)-6-[(2R,3S,4R,5R,6R)-4,5,6-trihydroxy-2-(hydroxymethyl)oxan-3-yl]oxyoxan-4-yl]oxy-5-hydroxy-6-(hydroxymethyl)oxan-4-yl]oxy-3,5-dihydroxy-6-(hydroxymethyl)oxan-4-yl]oxy-4-hydroxy-6-[(1R,2R)-1,2,3-trihydroxypropyl]oxane-2-carboxylic acid | |
---|---|---|
Source | PubChem | |
URL | https://pubchem.ncbi.nlm.nih.gov | |
Description | Data deposited in or computed by PubChem | |
InChI |
InChI=1S/C37H62N2O29/c1-9(45)38-17-11(47)3-37(36(58)59,67-29(17)19(49)12(48)4-40)68-31-22(52)15(7-43)63-35(26(31)56)65-28-18(39-10(2)46)33(61-13(5-41)20(28)50)66-30-21(51)14(6-42)62-34(25(30)55)64-27-16(8-44)60-32(57)24(54)23(27)53/h11-35,40-44,47-57H,3-8H2,1-2H3,(H,38,45)(H,39,46)(H,58,59)/t11-,12+,13+,14+,15+,16+,17+,18+,19+,20+,21-,22-,23+,24+,25+,26+,27+,28+,29+,30-,31-,32+,33-,34-,35-,37-/m0/s1 | |
Source | PubChem | |
URL | https://pubchem.ncbi.nlm.nih.gov | |
Description | Data deposited in or computed by PubChem | |
InChI Key |
IOAPDMZSLCJVCR-XWSXOIAXSA-N | |
Source | PubChem | |
URL | https://pubchem.ncbi.nlm.nih.gov | |
Description | Data deposited in or computed by PubChem | |
Canonical SMILES |
CC(=O)NC1C(CC(OC1C(C(CO)O)O)(C(=O)O)OC2C(C(OC(C2O)OC3C(C(OC(C3O)CO)OC4C(C(OC(C4O)OC5C(OC(C(C5O)O)O)CO)CO)O)NC(=O)C)CO)O)O | |
Source | PubChem | |
URL | https://pubchem.ncbi.nlm.nih.gov | |
Description | Data deposited in or computed by PubChem | |
Isomeric SMILES |
CC(=O)N[C@@H]1[C@H](C[C@@](O[C@H]1[C@@H]([C@@H](CO)O)O)(C(=O)O)O[C@H]2[C@H]([C@H](O[C@H]([C@@H]2O)O[C@@H]3[C@H]([C@@H](O[C@@H]([C@H]3O)CO)O[C@H]4[C@H]([C@H](O[C@H]([C@@H]4O)O[C@@H]5[C@H](O[C@H]([C@@H]([C@H]5O)O)O)CO)CO)O)NC(=O)C)CO)O)O | |
Source | PubChem | |
URL | https://pubchem.ncbi.nlm.nih.gov | |
Description | Data deposited in or computed by PubChem | |
Molecular Formula |
C37H62N2O29 | |
Source | PubChem | |
URL | https://pubchem.ncbi.nlm.nih.gov | |
Description | Data deposited in or computed by PubChem | |
DSSTOX Substance ID |
DTXSID50616695 | |
Record name | PUBCHEM_21670523 | |
Source | EPA DSSTox | |
URL | https://comptox.epa.gov/dashboard/DTXSID50616695 | |
Description | DSSTox provides a high quality public chemistry resource for supporting improved predictive toxicology. | |
Molecular Weight |
998.9 g/mol | |
Source | PubChem | |
URL | https://pubchem.ncbi.nlm.nih.gov | |
Description | Data deposited in or computed by PubChem | |
CAS No. |
52659-37-7 | |
Record name | PUBCHEM_21670523 | |
Source | EPA DSSTox | |
URL | https://comptox.epa.gov/dashboard/DTXSID50616695 | |
Description | DSSTox provides a high quality public chemistry resource for supporting improved predictive toxicology. | |
Descargo de responsabilidad e información sobre productos de investigación in vitro
Tenga en cuenta que todos los artículos e información de productos presentados en BenchChem están destinados únicamente con fines informativos. Los productos disponibles para la compra en BenchChem están diseñados específicamente para estudios in vitro, que se realizan fuera de organismos vivos. Los estudios in vitro, derivados del término latino "in vidrio", involucran experimentos realizados en entornos de laboratorio controlados utilizando células o tejidos. Es importante tener en cuenta que estos productos no se clasifican como medicamentos y no han recibido la aprobación de la FDA para la prevención, tratamiento o cura de ninguna condición médica, dolencia o enfermedad. Debemos enfatizar que cualquier forma de introducción corporal de estos productos en humanos o animales está estrictamente prohibida por ley. Es esencial adherirse a estas pautas para garantizar el cumplimiento de los estándares legales y éticos en la investigación y experimentación.