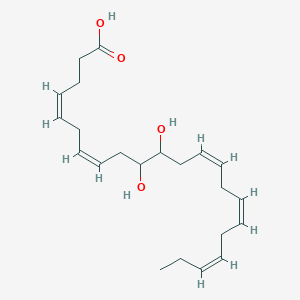
10,11-DiHDPE
Descripción general
Descripción
(±)10(11)-DiHDPA is a polyunsaturated fatty acid with multiple double bonds and hydroxyl groups. This compound is of significant interest due to its potential biological activities and applications in various scientific fields.
Aplicaciones Científicas De Investigación
Biochemical Pathways and Mechanisms
(±)10(11)-DiHDPA is primarily produced through the action of cytochrome P450 epoxygenases on DHA. It plays a significant role in the formation of specialized pro-resolving mediators (SPMs), which are crucial for resolving inflammation and promoting tissue repair. The biochemical pathways involving DiHDPA include:
- Conversion from DHA : DiHDPA is synthesized via enzymatic oxidation of DHA, leading to various dihydroxy metabolites that have distinct biological activities.
- Role in Inflammation : Studies indicate that DiHDPA can modulate inflammatory responses by influencing leukocyte recruitment and activation, thereby promoting the resolution phase of inflammation .
Pain Management
Recent studies have highlighted the potential of (±)10(11)-DiHDPA in pain management. For instance, a secondary analysis of plasma lipid mediators showed that higher levels of DHA-derived oxylipins, including DiHDPA, were associated with reduced headache frequency in patients undergoing dietary interventions . This suggests a therapeutic role for DiHDPA in managing chronic pain conditions.
Cardiovascular Health
(±)10(11)-DiHDPA has been implicated in cardiovascular health assessments. Quantification of plasma oxylipins, including DiHDPA, may assist in diagnosing and prognosing coronary artery disease (CAD). Elevated levels of specific oxylipins, including those derived from DHA, have been linked to improved cardiovascular outcomes .
Lipid Mediator Profiles
A comprehensive profiling of lipid mediators has shown significant alterations in the levels of (±)10(11)-DiHDPA under various physiological conditions. For example:
These findings suggest that DiHDPA may serve as a biomarker for metabolic and inflammatory conditions.
Case Studies
Several case studies have explored the impact of dietary interventions on DiHDPA levels:
- Dietary Omega-3 Supplementation : A study involving omega-3 supplementation demonstrated increased levels of (±)10(11)-DiHDPA in plasma samples, correlating with improved inflammatory markers and pain relief .
- Ischemic Injury Response : Research indicated that DiHDPA levels were significantly elevated in animal models following ischemic injury, suggesting its role in neuroprotection and recovery processes post-injury .
Mecanismo De Acción
Target of Action
10,11-DiHDPE is primarily produced from the action of the cytochrome P450 epoxygenase on docosahexaenoic acid (DHA) . This enzyme is the primary target of this compound and plays a crucial role in its formation .
Mode of Action
The interaction of this compound with its target, the cytochrome P450 epoxygenase, results in the conversion of DHA into this compound . This conversion is a key step in the compound’s mode of action .
Biochemical Pathways
The formation of this compound from DHA via the action of cytochrome P450 epoxygenase is part of the cytochrome P450 pathways . This pathway is crucial for the production of various bioactive lipid mediators, including this compound .
Result of Action
This compound has been shown to inhibit VEGF-induced angiogenesis in mice . This suggests that it may have anti-angiogenic properties, which could be beneficial in conditions where angiogenesis is a factor, such as cancer . Additionally, this compound may have anti-inflammatory and anti-tumor effects .
Análisis Bioquímico
Biochemical Properties
10,11-DiHDPE is involved in several biochemical reactions, primarily through its interactions with enzymes and proteins. One of the key enzymes it interacts with is cytochrome P450 epoxygenase, which catalyzes the conversion of DHA to this compound . This compound has been shown to inhibit vascular endothelial growth factor (VEGF)-induced angiogenesis, suggesting its potential role in anti-angiogenic and anti-tumor activities . Additionally, this compound may have anti-inflammatory effects, further highlighting its importance in biochemical pathways .
Cellular Effects
This compound exerts various effects on different cell types and cellular processes. It has been shown to influence cell signaling pathways, gene expression, and cellular metabolism. For instance, this compound inhibits VEGF-induced angiogenesis in endothelial cells, thereby affecting cell proliferation and migration . Moreover, it has been observed to modulate inflammatory responses in immune cells, potentially impacting cytokine production and immune cell function . These effects underscore the compound’s significance in regulating cellular activities and maintaining cellular homeostasis.
Molecular Mechanism
The molecular mechanism of this compound involves its interactions with various biomolecules, including enzymes and receptors. It binds to cytochrome P450 epoxygenase, facilitating the conversion of DHA to this compound . This compound also interacts with VEGF receptors, inhibiting their activation and subsequent signaling pathways . Additionally, this compound may modulate the activity of soluble epoxide hydrolase (sEH), an enzyme involved in the metabolism of epoxy fatty acids . These interactions contribute to the compound’s anti-angiogenic, anti-inflammatory, and anti-tumor effects.
Temporal Effects in Laboratory Settings
In laboratory settings, the effects of this compound have been studied over time to understand its stability, degradation, and long-term impact on cellular function. Studies have shown that this compound is relatively stable under experimental conditions, with minimal degradation over time . Long-term exposure to this compound has been observed to sustain its anti-angiogenic and anti-inflammatory effects, indicating its potential for prolonged therapeutic applications
Dosage Effects in Animal Models
The effects of this compound vary with different dosages in animal models. At lower doses, this compound has been shown to exhibit anti-angiogenic and anti-inflammatory properties without significant toxicity . At higher doses, potential adverse effects such as cytotoxicity and disruption of normal cellular functions may occur . These findings highlight the importance of determining optimal dosages for therapeutic applications to maximize benefits while minimizing potential risks.
Metabolic Pathways
This compound is involved in metabolic pathways related to the metabolism of DHA and other polyunsaturated fatty acids. It is produced through the action of cytochrome P450 epoxygenase on DHA and can be further metabolized by soluble epoxide hydrolase (sEH) to form dihydroxy derivatives . These metabolic pathways play crucial roles in regulating the levels of bioactive lipid mediators and maintaining cellular homeostasis. Additionally, this compound may influence metabolic flux and metabolite levels, further contributing to its biological effects .
Transport and Distribution
The transport and distribution of this compound within cells and tissues involve interactions with specific transporters and binding proteins. This compound can be transported across cell membranes through passive diffusion or facilitated transport mechanisms . Once inside the cells, this compound may bind to intracellular proteins, influencing its localization and accumulation . These interactions are essential for the compound’s bioavailability and its ability to exert its biological effects.
Subcellular Localization
The subcellular localization of this compound is critical for its activity and function. This compound can be localized to various cellular compartments, including the cytoplasm, mitochondria, and endoplasmic reticulum . The specific localization of this compound may be influenced by targeting signals or post-translational modifications that direct it to specific organelles . Understanding the subcellular localization of this compound is essential for elucidating its mechanism of action and optimizing its therapeutic potential.
Métodos De Preparación
Synthetic Routes and Reaction Conditions
The synthesis of (±)10(11)-DiHDPA typically involves the following steps:
Starting Material: The synthesis begins with a suitable polyunsaturated fatty acid precursor.
Isomerization: The double bonds are introduced and positioned through isomerization reactions, often using metal catalysts under controlled conditions to ensure the formation of the desired Z-isomers.
Industrial Production Methods
Industrial production of this compound may involve large-scale synthesis using similar methods but optimized for higher yields and purity. Techniques such as continuous flow reactors and advanced purification methods like high-performance liquid chromatography (HPLC) are employed to ensure the consistency and quality of the product.
Análisis De Reacciones Químicas
Types of Reactions
(±)10(11)-DiHDPA undergoes various chemical reactions, including:
Oxidation: The hydroxyl groups can be oxidized to form ketones or carboxylic acids.
Reduction: The double bonds can be reduced to single bonds using hydrogenation reactions.
Substitution: The hydroxyl groups can be substituted with other functional groups through nucleophilic substitution reactions.
Common Reagents and Conditions
Oxidation: Reagents such as potassium permanganate or chromium trioxide are used under acidic or basic conditions.
Reduction: Hydrogen gas in the presence of palladium or platinum catalysts is commonly used.
Substitution: Nucleophiles like halides or amines are used in the presence of suitable catalysts.
Major Products
Oxidation: Formation of ketones or carboxylic acids.
Reduction: Formation of saturated fatty acids.
Substitution: Formation of substituted fatty acids with various functional groups.
Comparación Con Compuestos Similares
(±)10(11)-DiHDPA can be compared with other polyunsaturated fatty acids such as:
Eicosapentaenoic Acid (EPA): Similar in structure but lacks the hydroxyl groups at the 10th and 11th positions.
Docosahexaenoic Acid (DHA): Contains more double bonds and is known for its role in brain health.
Arachidonic Acid: Another polyunsaturated fatty acid involved in inflammatory responses.
The unique presence of hydroxyl groups in (±)10(11)-DiHDPA distinguishes it from these similar compounds, potentially enhancing its biological activity and applications.
Actividad Biológica
(±)10(11)-DiHDPA, a dihydroxy derivative of docosahexaenoic acid (DHA), is gaining attention for its potential biological activities, particularly in the context of inflammation and neuroprotection. This article synthesizes findings from various studies to provide a comprehensive overview of the biological activity associated with (±)10(11)-DiHDPA, including its mechanisms of action, therapeutic potential, and relevant case studies.
Overview of (±)10(11)-DiHDPA
(±)10(11)-DiHDPA is formed through the enzymatic conversion of DHA via lipoxygenase (LOX) pathways. It is classified as an oxylipin, a group of bioactive lipids derived from polyunsaturated fatty acids (PUFAs). Oxylipins play critical roles in various physiological processes, including inflammation, pain modulation, and cell signaling.
-
Anti-inflammatory Effects :
- Studies suggest that (±)10(11)-DiHDPA exhibits anti-inflammatory properties by modulating cytokine release and inhibiting pro-inflammatory pathways. For instance, co-treatment with (±)10(11)-DiHDPA has been shown to prevent cytokine-induced reductions in neurogenesis and apoptosis in neuronal cells .
- Neuroprotective Properties :
- Biotransformation Pathways :
Table 1: Key Findings on Biological Activity
Case Study: Neuroprotective Effects in Major Depressive Disorder
A clinical trial involving 22 patients diagnosed with major depressive disorder explored the effects of EPA and DHA supplementation on plasma oxylipins levels, including (±)10(11)-DiHDPA. The study found that higher concentrations of these metabolites were associated with less severe depressive symptoms. This suggests that supplementation may enhance the levels of neuroprotective metabolites derived from PUFAs .
Propiedades
IUPAC Name |
(4Z,7Z,13Z,16Z,19Z)-10,11-dihydroxydocosa-4,7,13,16,19-pentaenoic acid | |
---|---|---|
Source | PubChem | |
URL | https://pubchem.ncbi.nlm.nih.gov | |
Description | Data deposited in or computed by PubChem | |
InChI |
InChI=1S/C22H34O4/c1-2-3-4-5-6-7-8-11-14-17-20(23)21(24)18-15-12-9-10-13-16-19-22(25)26/h3-4,6-7,10-15,20-21,23-24H,2,5,8-9,16-19H2,1H3,(H,25,26)/b4-3-,7-6-,13-10-,14-11-,15-12- | |
Source | PubChem | |
URL | https://pubchem.ncbi.nlm.nih.gov | |
Description | Data deposited in or computed by PubChem | |
InChI Key |
OAZUCYZBXHOCES-UQZHZJRSSA-N | |
Source | PubChem | |
URL | https://pubchem.ncbi.nlm.nih.gov | |
Description | Data deposited in or computed by PubChem | |
Canonical SMILES |
CCC=CCC=CCC=CCC(C(CC=CCC=CCCC(=O)O)O)O | |
Source | PubChem | |
URL | https://pubchem.ncbi.nlm.nih.gov | |
Description | Data deposited in or computed by PubChem | |
Isomeric SMILES |
CC/C=C\C/C=C\C/C=C\CC(C(C/C=C\C/C=C\CCC(=O)O)O)O | |
Source | PubChem | |
URL | https://pubchem.ncbi.nlm.nih.gov | |
Description | Data deposited in or computed by PubChem | |
Molecular Formula |
C22H34O4 | |
Source | PubChem | |
URL | https://pubchem.ncbi.nlm.nih.gov | |
Description | Data deposited in or computed by PubChem | |
Molecular Weight |
362.5 g/mol | |
Source | PubChem | |
URL | https://pubchem.ncbi.nlm.nih.gov | |
Description | Data deposited in or computed by PubChem | |
Retrosynthesis Analysis
AI-Powered Synthesis Planning: Our tool employs the Template_relevance Pistachio, Template_relevance Bkms_metabolic, Template_relevance Pistachio_ringbreaker, Template_relevance Reaxys, Template_relevance Reaxys_biocatalysis model, leveraging a vast database of chemical reactions to predict feasible synthetic routes.
One-Step Synthesis Focus: Specifically designed for one-step synthesis, it provides concise and direct routes for your target compounds, streamlining the synthesis process.
Accurate Predictions: Utilizing the extensive PISTACHIO, BKMS_METABOLIC, PISTACHIO_RINGBREAKER, REAXYS, REAXYS_BIOCATALYSIS database, our tool offers high-accuracy predictions, reflecting the latest in chemical research and data.
Strategy Settings
Precursor scoring | Relevance Heuristic |
---|---|
Min. plausibility | 0.01 |
Model | Template_relevance |
Template Set | Pistachio/Bkms_metabolic/Pistachio_ringbreaker/Reaxys/Reaxys_biocatalysis |
Top-N result to add to graph | 6 |
Feasible Synthetic Routes
Q1: The study mentions that (+/-)-10,11-dihydroxy-4Z,7Z,13Z,16Z,19Z-docosapentaenoic acid (10,11-DiHDPE) levels were found to be elevated in the plasma of individuals taking celecoxib. What is the potential significance of this finding?
A1: The study suggests that celecoxib, while aiming to inhibit COX2 and reduce pro-inflammatory prostaglandin E2 (PGE2), may unintentionally stimulate other enzymatic pathways involved in arachidonic acid metabolism. [] This can lead to the increased production of metabolites like this compound, which are associated with hypertension. [] This finding highlights the complexity of arachidonic acid pathways and the potential for unintended consequences when using selective COX2 inhibitors like celecoxib. Further research is needed to fully understand the long-term implications of elevated this compound levels in individuals taking celecoxib.
Descargo de responsabilidad e información sobre productos de investigación in vitro
Tenga en cuenta que todos los artículos e información de productos presentados en BenchChem están destinados únicamente con fines informativos. Los productos disponibles para la compra en BenchChem están diseñados específicamente para estudios in vitro, que se realizan fuera de organismos vivos. Los estudios in vitro, derivados del término latino "in vidrio", involucran experimentos realizados en entornos de laboratorio controlados utilizando células o tejidos. Es importante tener en cuenta que estos productos no se clasifican como medicamentos y no han recibido la aprobación de la FDA para la prevención, tratamiento o cura de ninguna condición médica, dolencia o enfermedad. Debemos enfatizar que cualquier forma de introducción corporal de estos productos en humanos o animales está estrictamente prohibida por ley. Es esencial adherirse a estas pautas para garantizar el cumplimiento de los estándares legales y éticos en la investigación y experimentación.