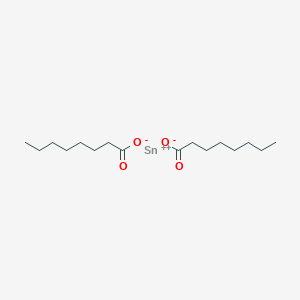
Stannous dioctanoate
Descripción general
Descripción
Stannous dioctanoate (C₁₆H₃₀O₄Sn), also known as tin(II) octanoate or stannous octoate, is an organotin compound with the CAS registry number 1912-83-0 . It is classified as an anionic surfactant and carboxylate salt, widely utilized in industrial and biomedical applications. Its primary roles include serving as a catalyst in polymerization reactions, such as the synthesis of polyurethane foams and biodegradable polyesters like poly(lactic-co-glycolic acid) (PLGA) . In polyurethane production, this compound optimizes the balance between gelation and blowing reactions, making it indispensable for flexible foam manufacturing . However, its toxicity in biomedical contexts (e.g., PLGA microspheres for drug delivery) has prompted substitution with less toxic alternatives, such as zinc dioctanoate .
Métodos De Preparación
Anhydride Method
The anhydride method is a widely documented approach for synthesizing stannous dioctanoate. This technique involves reacting stannous oxide (SnO) with 2-ethylhexanoic anhydride under controlled conditions.
Reaction Mechanism and Conditions
The reaction proceeds via nucleophilic acyl substitution, where stannous oxide acts as a Lewis acid to facilitate the cleavage of the anhydride bond. The general stoichiometry is:
Key parameters include:
Industrial Refinements
A modified anhydride method employs vacuum distillation (105–145°C) to remove water and unreacted acid, achieving >95% purity . The addition of antioxidants (e.g., hindered phenols) further stabilizes the product against oxidative degradation .
Table 1: Optimal Parameters for Anhydride Method
Parameter | Range | Impact on Yield/Purity |
---|---|---|
Temperature | 120–140°C | Higher temps accelerate reaction but risk oxidation |
Stirring Speed | 300–400 rpm | Ensures homogeneous mixing |
Antioxidant Concentration | 0.1–0.5 wt% | Prevents Sn(II) → Sn(IV) oxidation |
Vacuum Pressure | 10–15 mmHg | Removes volatiles efficiently |
Double Decomposition Method
This method leverages metathesis reactions between stannous chloride (SnCl<sub>2</sub>) and sodium 2-ethylhexanoate (NaC<sub>7</sub>H<sub>15</sub>COO).
Reaction Pathway
Challenges and Solutions
-
Byproduct Removal : Sodium chloride precipitates are filtered, but residual NaCl can contaminate the product. Industrial protocols use solvent extraction (e.g., toluene) to isolate this compound .
-
pH Control : Maintaining a pH of 4.5–5.5 prevents hydrolysis of SnCl<sub>2</sub> into Sn(OH)<sub>2</sub> .
Table 2: Yield Optimization in Double Decomposition
Variable | Optimal Value | Effect |
---|---|---|
Molar Ratio (SnCl<sub>2</sub>:NaC<sub>7</sub>H<sub>15</sub>COO) | 1:2.1 | Excess Na salt drives reaction |
Solvent | Toluene | High partition coefficient |
Reaction Time | 30–45 minutes | Prolonged time risks side reactions |
Electrochemical Synthesis
The electrochemical method offers a solvent-free route, utilizing tin metal as the anode in an electrolytic cell.
Mechanism
At the anode:
At the cathode:
Tin ions react with 2-ethylhexanoic acid in the electrolyte:
Industrial Scalability
-
Current Density : 100–120 A/m² at the anode and 800–1000 A/m² at the cathode.
-
Electrolyte Composition : Sodium chloride (5–10 wt%) with 2-ethylhexanoic acid.
Table 3: Electrochemical Process Parameters
Parameter | Value | Note |
---|---|---|
Temperature | 60–70°C | Enhances ion mobility |
Cell Voltage | 3–4 V | Minimizes side reactions |
Acid Concentration | 20–25 wt% | Balances conductivity and cost |
One-Step Synthesis (Patent CN1180691A)
A novel one-step method replaces sodium hydroxide with sodium carbonate (Na<sub>2</sub>CO<sub>3</sub>), reducing costs and simplifying purification.
Reaction Overview
Key Innovations
-
CO<sub>2</sub> Atmosphere : Retained to inhibit oxidation, supplemented with argon .
-
Antioxidant Additives : "No. 1 oxidation inhibitor" (proprietary) enhances stability .
-
Mol Ratio Optimization : 2-ethylhexanoic acid : SnCl<sub>2</sub> : Na<sub>2</sub>CO<sub>3</sub> = 1 : 0.4–0.7 : 0.45–0.75 .
Table 4: One-Step Method Performance Metrics
Metric | Value | Advantage |
---|---|---|
Tin Conversion Efficiency | 92–95% | Reduces raw material waste |
Production Cost | 30% lower than NaOH-based methods | Economical for scale-up |
Purity | 98–99% | Competes with imported products |
Comparative Analysis of Methods
Yield and Purity
-
Anhydride Method : Highest purity (99%) but requires expensive anhydride .
-
Double Decomposition : Moderate yield (85–90%) with NaCl contamination risks .
-
Electrochemical : Eco-friendly but energy-intensive.
-
One-Step Synthesis : Balances cost and efficiency, ideal for industrialization .
Industrial Adoption
Análisis De Reacciones Químicas
Types of Reactions: Stannous dioctanoate undergoes various chemical reactions, including:
Oxidation: It can be oxidized to form tin(IV) compounds.
Reduction: In basic solutions, this compound can reduce bismuth(III) to metallic bismuth.
Substitution: It can participate in substitution reactions with other organic compounds.
Common Reagents and Conditions:
Oxidation: Oxygen or other oxidizing agents under ambient conditions.
Reduction: Basic solutions such as sodium hydroxide.
Substitution: Organic solvents and appropriate reactants depending on the desired substitution product.
Major Products:
Oxidation: Tin(IV) compounds.
Reduction: Metallic bismuth.
Substitution: Various organotin compounds depending on the reactants used.
Aplicaciones Científicas De Investigación
Chemical Catalysis
Ring-Opening Polymerization (ROP)
Stannous dioctanoate is widely recognized as an effective catalyst for the ring-opening polymerization of lactides and caprolactones. These reactions are crucial for producing biodegradable polymers used in various applications, such as packaging and medical devices. The compound facilitates the polymerization process by providing a mechanism that reduces the energy barrier for the reaction, leading to higher reaction rates and improved yields of polylactides .
Comparison with Other Catalysts
In studies comparing this compound with other tin-based catalysts, it has been shown to exhibit lower racemization rates during polymerization, making it a preferred choice for synthesizing high-purity polylactides. This characteristic is particularly important in applications where optical purity affects the material properties .
Biomedical Applications
Synthesis of Biocompatible Materials
this compound plays a critical role in synthesizing biocompatible materials for medical applications. Its ability to catalyze polymerization reactions is leveraged to create materials suitable for drug delivery systems and biodegradable implants. The low toxicity of tin(II) ions further enhances its appeal for use in biomedical contexts, as it poses minimal risk during human exposure .
Case Study: Drug Delivery Systems
A notable application involves using this compound in the preparation of liposomes for targeted drug delivery. Research has demonstrated that liposomes formed using this catalyst can encapsulate antibiotics effectively, improving their stability and bioavailability while minimizing side effects in animal models .
Industrial Applications
Polyurethane Production
In the polyurethane industry, this compound serves as a catalyst that accelerates the cross-linking reactions between polyether polyols and diisocyanates. This application is vital for producing flexible foams used in furniture, automotive seating, and insulation materials. The compound's efficiency ensures that the foaming reaction and cross-linking processes are well-balanced, resulting in products with desirable mechanical properties .
Table: Summary of Industrial Applications
Application Area | Specific Use | Benefits |
---|---|---|
Polymer Chemistry | Ring-opening polymerization of lactides | Produces biodegradable polymers |
Biomedical Engineering | Synthesis of drug delivery systems | Enhances stability and bioavailability |
Polyurethane Manufacturing | Catalyst in foam production | Improves mechanical properties |
Safety and Environmental Considerations
While this compound is generally considered safe for use in controlled environments, it is essential to adhere to safety guidelines due to potential toxicity associated with tin compounds. Regulatory bodies have evaluated its use in food stabilization and medical applications, confirming that when used appropriately, it poses minimal risks to human health .
Mecanismo De Acción
Stannous dioctanoate acts as a catalyst by facilitating the formation of reactive intermediates in polymerization reactions. The tin(II) ion interacts with the monomers, promoting the opening of the ring structure and enabling the polymerization process . The molecular targets include the carbonyl groups of the monomers, and the pathways involved are primarily related to the coordination and insertion mechanisms .
Comparación Con Compuestos Similares
Comparison with Similar Stannous Compounds
Stannous compounds share the Sn²⁺ ion but differ markedly in structure, applications, and performance. Below is a comparative analysis of stannous dioctanoate with analogous compounds:
Stannous Fluoride (SnF₂)
- Applications : Primarily used in dental care products (toothpastes, mouth rinses) for anti-erosive and anti-caries effects.
- Efficacy: SnF₂ demonstrates superior enamel protection compared to NaF or AmF due to stannous ions' ability to form acid-resistant tin-oxide layers and inhibit bacterial metabolism . However, in toothpaste formulations, stannous ions may adsorb onto silica abrasives, reducing bioavailability .
- Toxicity : Generally recognized as safe (GRAS) in regulated concentrations for oral use.
Stannous Chloride (SnCl₂)
- Applications : Employed in dental rinses, tin plating, and as a reducing agent in chemical synthesis .
- Efficacy : Exhibits anti-erosive properties in dental care, particularly when combined with fluorides (e.g., AmF/NaF), enhancing protection by 20–30% compared to standalone use .
- Toxicity : Corrosive and unstable in aqueous solutions, necessitating stabilizers for clinical applications (e.g., lyophilized kits for radiolabeling red blood cells) .
Stannous Methanesulfonate (Sn(CH₃SO₃)₂)
- Applications : Emerging in niche industrial processes, including electroplating and catalysis.
- Market Data: The global stannous methanesulfonate market is projected to grow at a CAGR of 4.2% (2024–2031), driven by demand in electronics and specialty chemicals .
- Advantage: Offers higher solubility in polar solvents compared to this compound.
Dibutyltin Dioctanoate (C₃₂H₆₀O₄Sn)
- Applications : A dialkyltin compound used as a heat stabilizer in PVC production and a catalyst in polyesters.
- Key Difference: Unlike this compound, dibutyltin derivatives exhibit higher thermal stability but greater environmental persistence, raising regulatory concerns .
Stannous Oxalate (SnC₂O₄)
- Applications: Limited to laboratory-scale synthesis and specialty coatings.
- Safety Profile: Not classified as a persistent, bioaccumulative, or toxic (PBT) substance, making it environmentally preferable to organotin catalysts like this compound .
Comparative Performance Data
Research Findings and Limitations
- Formulation Challenges: Stannous compounds in toothpaste (e.g., SnF₂) underperform compared to mouth rinses due to interactions with excipients like silica, which adsorb stannous ions .
- Synergistic Effects: Combining stannous chloride with AmF/NaF enhances anti-erosive efficacy by 25–30%, suggesting multi-agent formulations maximize performance .
- Toxicity Trade-offs: While this compound is ideal for industrial catalysis, its toxicity in PLGA synthesis necessitates alternatives like zinc dioctanoate for biomedical safety .
Actividad Biológica
Stannous dioctanoate, also known as stannous octanoate, is an organotin compound with the chemical formula . It has garnered interest in various fields due to its biological activities, particularly in antioxidant properties and its role as a catalyst in polymerization processes. This article reviews the biological activity of this compound, supported by data tables, case studies, and research findings.
This compound is a pale yellow liquid that is soluble in organic solvents but insoluble in water. It is primarily synthesized through the reaction of stannous oxide with isooctanoic acid under controlled conditions, which allows for the production of high-purity this compound .
Antioxidant Activity
Research has demonstrated that this compound exhibits significant antioxidant activity. This property is attributed to its ability to scavenge free radicals, which can lead to oxidative stress and cellular damage. A comparative study evaluated the antioxidant activities of various organotin complexes using the DPPH (1,1-diphenyl-2-picrylhydrazyl) and CUPRAC (cupric ion reducing antioxidant capacity) methods. The results indicated that organotin complexes, including those derived from this compound, showed higher inhibition rates than their corresponding ligands .
Table 1: Antioxidant Activity of this compound and Related Compounds
Compound | DPPH Inhibition (%) | CUPRAC Activity (%) |
---|---|---|
Ligand (IAS) | 30 | 25 |
This compound | 75 | 70 |
Di-methyltin-di-IAS | 85 | 80 |
Phenyltin Complex | 60 | 55 |
The data indicates that this compound significantly enhances antioxidant capacity compared to its ligand counterpart, highlighting its potential therapeutic applications in oxidative stress-related conditions.
The mechanism by which this compound exerts its biological effects involves several pathways:
- Radical Scavenging : The tin moiety can donate electrons to free radicals, stabilizing them and preventing cellular damage.
- Metal Ion Interaction : The presence of tin may facilitate interactions with various biomolecules, enhancing their stability and activity.
- Catalytic Role : As a catalyst in polymerization reactions, this compound can influence the molecular weight and properties of polymers, which may indirectly affect biological systems .
Case Studies
- Polymerization Studies : In a study investigating the use of this compound as a catalyst for lactide polymerization, it was found that the compound significantly improved reaction rates and polymer characteristics. The study highlighted its effectiveness in producing polylactides with narrow molecular weight distributions .
- Toxicity Assessments : While this compound shows promising biological activities, toxicity studies are crucial. Research has indicated that organotin compounds can exhibit cytotoxic effects at high concentrations. Therefore, understanding the dose-response relationship is essential for safe application .
- Antimicrobial Properties : Additional studies have explored the antimicrobial effects of stannous compounds against various pathogens. This compound demonstrated inhibitory effects against both gram-positive and gram-negative bacteria, suggesting its potential as an antimicrobial agent .
Q & A
Basic Research Questions
Q. What experimental models are most suitable for evaluating the catalytic efficiency of stannous dioctanoate in polymerization reactions?
- Methodological Approach: Use controlled polymerization experiments (e.g., ring-opening polymerization of lactides) with Sn(Oct)₂ as a catalyst. Monitor reaction kinetics via gel permeation chromatography (GPC) and nuclear magnetic resonance (NMR) to assess molecular weight distribution and conversion rates. Include control groups with alternative catalysts (e.g., zinc-based compounds) for comparative analysis .
- Key Variables: Temperature (typically 120–180°C), monomer-to-catalyst ratio (e.g., 1,000:1), and solvent polarity.
Q. How can researchers characterize the coordination chemistry of this compound in solution?
- Methodological Approach: Employ spectroscopic techniques such as Fourier-transform infrared spectroscopy (FTIR) to identify Sn-O bonding patterns and X-ray absorption spectroscopy (XAS) to study local coordination environments. Pair with computational modeling (DFT calculations) to validate proposed structures .
Q. What standardized protocols exist for assessing the stability of this compound in aqueous environments?
- Methodological Approach: Conduct accelerated aging studies under varying pH (2–10) and temperature (25–60°C) conditions. Use inductively coupled plasma mass spectrometry (ICP-MS) to quantify Sn²⁺ ion release over time, correlating with changes in catalytic activity .
Advanced Research Questions
Q. How do conflicting results in this compound’s anti-erosive efficacy (e.g., dental studies) arise, and how can they be reconciled?
- Case Study: A 2022 study found Sn(Oct)₂ combined with fluoride (F+Sn) reduced bovine enamel erosion by 40% versus fluoride alone (25%). Contradictions with earlier studies may stem from differences in Sn²⁺ concentration (15,000 ppm vs. <5,000 ppm) or pH (4.5 vs. neutral) .
- Resolution Strategy: Meta-regression analysis to isolate variables (e.g., pH, Sn²⁺ concentration) across studies. Use standardized erosion models (e.g., 20-day cycling protocols) to minimize variability .
Q. What mechanisms explain this compound’s dual role as a catalyst and bioactive agent?
- Hypothesis Testing: In polymer chemistry, Sn(Oct)₂’s Lewis acidity facilitates monomer activation. In biomedical contexts, Sn²⁺ ions may inhibit bacterial adhesion via electrostatic interactions. Design crossover experiments to test both roles in a single system (e.g., Sn(Oct)₂ in antimicrobial polymer coatings) .
Q. How can researchers optimize experimental designs to address Sn(Oct)₂’s oxygen sensitivity in catalytic applications?
- Methodological Answer: Implement glovebox protocols for catalyst handling and reaction initiation. Use in situ Raman spectroscopy to monitor oxidation states during polymerization. Compare anaerobic vs. aerobic conditions to quantify oxygen’s impact on reaction yields .
Q. Methodological Challenges and Solutions
Q. What statistical approaches are recommended for analyzing dose-response relationships in Sn(Oct)₂ studies?
- Guidance: Use ANOVA with post-hoc Tukey tests for multi-group comparisons (e.g., varying Sn²⁺ concentrations). For non-linear responses, apply generalized additive models (GAMs) or segmented regression .
Q. How can researchers mitigate batch-to-batch variability in this compound synthesis?
- Best Practices: Standardize precursor purification (e.g., vacuum distillation of octanoic acid) and validate synthesis via Karl Fischer titration (water content <50 ppm). Include batch identifiers in datasets for retrospective analysis .
Q. Cross-Disciplinary Applications
Q. What novel applications exist for this compound in energy storage materials?
- Emerging Research: Sn(Oct)₂-derived tin oxide (SnO₂) composites show promise in lithium-ion battery anodes. Use sol-gel synthesis to control SnO₂ morphology and test electrochemical performance via cyclic voltammetry .
Q. How can computational chemistry accelerate the development of Sn(Oct)₂-based catalysts?
Propiedades
IUPAC Name |
octanoate;tin(2+) | |
---|---|---|
Source | PubChem | |
URL | https://pubchem.ncbi.nlm.nih.gov | |
Description | Data deposited in or computed by PubChem | |
InChI |
InChI=1S/2C8H16O2.Sn/c2*1-2-3-4-5-6-7-8(9)10;/h2*2-7H2,1H3,(H,9,10);/q;;+2/p-2 | |
Source | PubChem | |
URL | https://pubchem.ncbi.nlm.nih.gov | |
Description | Data deposited in or computed by PubChem | |
InChI Key |
JQZRVMZHTADUSY-UHFFFAOYSA-L | |
Source | PubChem | |
URL | https://pubchem.ncbi.nlm.nih.gov | |
Description | Data deposited in or computed by PubChem | |
Canonical SMILES |
CCCCCCCC(=O)[O-].CCCCCCCC(=O)[O-].[Sn+2] | |
Source | PubChem | |
URL | https://pubchem.ncbi.nlm.nih.gov | |
Description | Data deposited in or computed by PubChem | |
Molecular Formula |
C16H30O4Sn | |
Source | PubChem | |
URL | https://pubchem.ncbi.nlm.nih.gov | |
Description | Data deposited in or computed by PubChem | |
Related CAS |
124-07-2 (Parent) | |
Record name | Octanoic acid, tin(2+) salt (2:1) | |
Source | ChemIDplus | |
URL | https://pubchem.ncbi.nlm.nih.gov/substance/?source=chemidplus&sourceid=0001912830 | |
Description | ChemIDplus is a free, web search system that provides access to the structure and nomenclature authority files used for the identification of chemical substances cited in National Library of Medicine (NLM) databases, including the TOXNET system. | |
DSSTOX Substance ID |
DTXSID10890550 | |
Record name | Octanoic acid, tin(2+) salt (2:1) | |
Source | EPA DSSTox | |
URL | https://comptox.epa.gov/dashboard/DTXSID10890550 | |
Description | DSSTox provides a high quality public chemistry resource for supporting improved predictive toxicology. | |
Molecular Weight |
405.1 g/mol | |
Source | PubChem | |
URL | https://pubchem.ncbi.nlm.nih.gov | |
Description | Data deposited in or computed by PubChem | |
CAS No. |
1912-83-0 | |
Record name | Octanoic acid, tin(2+) salt (2:1) | |
Source | ChemIDplus | |
URL | https://pubchem.ncbi.nlm.nih.gov/substance/?source=chemidplus&sourceid=0001912830 | |
Description | ChemIDplus is a free, web search system that provides access to the structure and nomenclature authority files used for the identification of chemical substances cited in National Library of Medicine (NLM) databases, including the TOXNET system. | |
Record name | Octanoic acid, tin(2+) salt (2:1) | |
Source | EPA Chemicals under the TSCA | |
URL | https://www.epa.gov/chemicals-under-tsca | |
Description | EPA Chemicals under the Toxic Substances Control Act (TSCA) collection contains information on chemicals and their regulations under TSCA, including non-confidential content from the TSCA Chemical Substance Inventory and Chemical Data Reporting. | |
Record name | Octanoic acid, tin(2+) salt (2:1) | |
Source | EPA DSSTox | |
URL | https://comptox.epa.gov/dashboard/DTXSID10890550 | |
Description | DSSTox provides a high quality public chemistry resource for supporting improved predictive toxicology. | |
Record name | Tin di(octanoate) | |
Source | European Chemicals Agency (ECHA) | |
URL | https://echa.europa.eu/substance-information/-/substanceinfo/100.016.023 | |
Description | The European Chemicals Agency (ECHA) is an agency of the European Union which is the driving force among regulatory authorities in implementing the EU's groundbreaking chemicals legislation for the benefit of human health and the environment as well as for innovation and competitiveness. | |
Explanation | Use of the information, documents and data from the ECHA website is subject to the terms and conditions of this Legal Notice, and subject to other binding limitations provided for under applicable law, the information, documents and data made available on the ECHA website may be reproduced, distributed and/or used, totally or in part, for non-commercial purposes provided that ECHA is acknowledged as the source: "Source: European Chemicals Agency, http://echa.europa.eu/". Such acknowledgement must be included in each copy of the material. ECHA permits and encourages organisations and individuals to create links to the ECHA website under the following cumulative conditions: Links can only be made to webpages that provide a link to the Legal Notice page. | |
Retrosynthesis Analysis
AI-Powered Synthesis Planning: Our tool employs the Template_relevance Pistachio, Template_relevance Bkms_metabolic, Template_relevance Pistachio_ringbreaker, Template_relevance Reaxys, Template_relevance Reaxys_biocatalysis model, leveraging a vast database of chemical reactions to predict feasible synthetic routes.
One-Step Synthesis Focus: Specifically designed for one-step synthesis, it provides concise and direct routes for your target compounds, streamlining the synthesis process.
Accurate Predictions: Utilizing the extensive PISTACHIO, BKMS_METABOLIC, PISTACHIO_RINGBREAKER, REAXYS, REAXYS_BIOCATALYSIS database, our tool offers high-accuracy predictions, reflecting the latest in chemical research and data.
Strategy Settings
Precursor scoring | Relevance Heuristic |
---|---|
Min. plausibility | 0.01 |
Model | Template_relevance |
Template Set | Pistachio/Bkms_metabolic/Pistachio_ringbreaker/Reaxys/Reaxys_biocatalysis |
Top-N result to add to graph | 6 |
Feasible Synthetic Routes
Descargo de responsabilidad e información sobre productos de investigación in vitro
Tenga en cuenta que todos los artículos e información de productos presentados en BenchChem están destinados únicamente con fines informativos. Los productos disponibles para la compra en BenchChem están diseñados específicamente para estudios in vitro, que se realizan fuera de organismos vivos. Los estudios in vitro, derivados del término latino "in vidrio", involucran experimentos realizados en entornos de laboratorio controlados utilizando células o tejidos. Es importante tener en cuenta que estos productos no se clasifican como medicamentos y no han recibido la aprobación de la FDA para la prevención, tratamiento o cura de ninguna condición médica, dolencia o enfermedad. Debemos enfatizar que cualquier forma de introducción corporal de estos productos en humanos o animales está estrictamente prohibida por ley. Es esencial adherirse a estas pautas para garantizar el cumplimiento de los estándares legales y éticos en la investigación y experimentación.