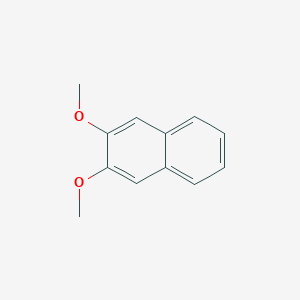
2,3-Dimethoxynaphthalene
Descripción general
Descripción
2,3-Dimethoxynaphthalene is a chemical compound with the molecular formula C12H12O2 . It has an average mass of 188.223 Da and a monoisotopic mass of 188.083725 Da . It is used as a pharmaceutical intermediate .
Molecular Structure Analysis
The molecular structure of 2,3-Dimethoxynaphthalene consists of 12 carbon atoms, 12 hydrogen atoms, and 2 oxygen atoms . The structure can be represented as C10H6(OCH3)2 .
Physical And Chemical Properties Analysis
2,3-Dimethoxynaphthalene has a density of 1.1±0.1 g/cm3, a boiling point of 295.9±13.0 °C at 760 mmHg, and a vapour pressure of 0.0±0.6 mmHg at 25°C . It has an enthalpy of vaporization of 51.4±3.0 kJ/mol and a flash point of 120.7±19.4 °C . The index of refraction is 1.584 .
Aplicaciones Científicas De Investigación
Pharmaceutical Applications
2,3-Dimethoxynaphthalene is utilized as an intermediate in pharmaceutical research. Its structure is being explored for pharmacological activities, including potential anti-cancer properties. Studies have synthesized naphthalene derivatives to evaluate their effects on triple-negative breast cancer (TNBC) and STAT3 signaling pathways, indicating its significance in cancer research .
Chromatography and Mass Spectrometry
This compound is also relevant in chromatography or mass spectrometry applications. It can be used for sample manipulation during these processes, which are crucial for separating and analyzing complex mixtures in scientific research .
Synthesis of Derivatives
Research has been conducted on the synthesis of various naphthalene derivatives, including 2,3-Dimethoxynaphthalene. These derivatives have applications across different fields of chemistry and materials science .
Safety and Hazards
When handling 2,3-Dimethoxynaphthalene, it’s important to wear personal protective equipment and ensure adequate ventilation . Avoid contact with skin, eyes, or clothing, and avoid ingestion and inhalation . In case of accidental release, avoid dust formation and do not let the product enter drains .
Relevant Papers
One relevant paper is “Nitration of 2,3-dimethoxynaphthalene” published in the Journal of the Chemical Society C: Organic . The paper discusses the nitration of 2,3-dimethoxynaphthalene leading to three mononitro-products, one dinitro-product, and one 1,2-naphthaquinone .
Mecanismo De Acción
Target of Action
As a derivative of naphthoquinone , it may interact with similar biological targets, such as enzymes involved in cellular respiration and redox reactions.
Mode of Action
2,3-Dimethoxynaphthalene is a redox-cycling agent . It induces the formation of intracellular superoxide anion, a type of reactive oxygen species (ROS). The interaction of 2,3-Dimethoxynaphthalene with its targets likely involves electron transfer reactions, leading to changes in the redox state of the cell.
Biochemical Pathways
Given its role as a redox-cycling agent , it can be inferred that it may influence pathways involving oxidative stress and cellular respiration.
Pharmacokinetics
It is reported to have high gastrointestinal absorption and is permeable to the blood-brain barrier . Its lipophilicity, as indicated by the Log Po/w value, suggests that it may readily cross cell membranes .
Result of Action
The molecular and cellular effects of 2,3-Dimethoxynaphthalene’s action depend on its concentration . At certain concentrations, it can induce cell proliferation, while at others, it can trigger apoptosis (programmed cell death) or necrosis (cell death due to damage) . These effects are likely due to the generation of ROS and the subsequent oxidative stress.
Propiedades
IUPAC Name |
2,3-dimethoxynaphthalene | |
---|---|---|
Source | PubChem | |
URL | https://pubchem.ncbi.nlm.nih.gov | |
Description | Data deposited in or computed by PubChem | |
InChI |
InChI=1S/C12H12O2/c1-13-11-7-9-5-3-4-6-10(9)8-12(11)14-2/h3-8H,1-2H3 | |
Source | PubChem | |
URL | https://pubchem.ncbi.nlm.nih.gov | |
Description | Data deposited in or computed by PubChem | |
InChI Key |
XYRPWXOJBNGTMX-UHFFFAOYSA-N | |
Source | PubChem | |
URL | https://pubchem.ncbi.nlm.nih.gov | |
Description | Data deposited in or computed by PubChem | |
Canonical SMILES |
COC1=CC2=CC=CC=C2C=C1OC | |
Source | PubChem | |
URL | https://pubchem.ncbi.nlm.nih.gov | |
Description | Data deposited in or computed by PubChem | |
Molecular Formula |
C12H12O2 | |
Source | PubChem | |
URL | https://pubchem.ncbi.nlm.nih.gov | |
Description | Data deposited in or computed by PubChem | |
DSSTOX Substance ID |
DTXSID70345470 | |
Record name | 2,3-Dimethoxynaphthalene | |
Source | EPA DSSTox | |
URL | https://comptox.epa.gov/dashboard/DTXSID70345470 | |
Description | DSSTox provides a high quality public chemistry resource for supporting improved predictive toxicology. | |
Molecular Weight |
188.22 g/mol | |
Source | PubChem | |
URL | https://pubchem.ncbi.nlm.nih.gov | |
Description | Data deposited in or computed by PubChem | |
Product Name |
2,3-Dimethoxynaphthalene | |
CAS RN |
10103-06-7 | |
Record name | 2,3-Dimethoxynaphthalene | |
Source | EPA DSSTox | |
URL | https://comptox.epa.gov/dashboard/DTXSID70345470 | |
Description | DSSTox provides a high quality public chemistry resource for supporting improved predictive toxicology. | |
Retrosynthesis Analysis
AI-Powered Synthesis Planning: Our tool employs the Template_relevance Pistachio, Template_relevance Bkms_metabolic, Template_relevance Pistachio_ringbreaker, Template_relevance Reaxys, Template_relevance Reaxys_biocatalysis model, leveraging a vast database of chemical reactions to predict feasible synthetic routes.
One-Step Synthesis Focus: Specifically designed for one-step synthesis, it provides concise and direct routes for your target compounds, streamlining the synthesis process.
Accurate Predictions: Utilizing the extensive PISTACHIO, BKMS_METABOLIC, PISTACHIO_RINGBREAKER, REAXYS, REAXYS_BIOCATALYSIS database, our tool offers high-accuracy predictions, reflecting the latest in chemical research and data.
Strategy Settings
Precursor scoring | Relevance Heuristic |
---|---|
Min. plausibility | 0.01 |
Model | Template_relevance |
Template Set | Pistachio/Bkms_metabolic/Pistachio_ringbreaker/Reaxys/Reaxys_biocatalysis |
Top-N result to add to graph | 6 |
Feasible Synthetic Routes
Q & A
Q1: What is the structural characterization of 2,3-Dimethoxynaphthalene?
A1: 2,3-Dimethoxynaphthalene is an organic compound with the molecular formula C12H12O2. It has a molecular weight of 188.22 g/mol. Spectroscopic data, including FT-IR, FT-Raman, and 13C NMR, have been used to characterize its structure. [, ]
Q2: How does the structure of 2,3-Dimethoxynaphthalene relate to its activity?
A2: Research on structurally similar compounds, specifically 1,2,3,4-tetraoxygenated naphthalene derivatives, suggests that net lipophilicity, hydrolytic stability, and ring substitution play significant roles in determining their biological activity. [] This insight suggests that modifications to the 2,3-Dimethoxynaphthalene structure could be explored to optimize its properties for specific applications.
Q3: What is known about the photochemical properties of 2,3-Dimethoxynaphthalene?
A3: 2,3-Dimethoxynaphthalene forms charge-transfer complexes with π-acceptors like 1,2-dicyanoethylenes. Upon irradiation, these complexes undergo photochemical reactions leading to isomerization of the olefins. The photostationary state depends on the specific donor-acceptor pair and their respective absorption spectra. []
Q4: Can you elaborate on the electron transfer dynamics observed in systems containing 2,3-Dimethoxynaphthalene?
A4: Studies utilizing 2,3-Dimethoxynaphthalene as an electron donor paired with 2,3-dicyanonaphthalene as an acceptor, bridged by n-norbornadiene, have shown a transition from adiabatic to nonadiabatic electron transfer depending on the bridge length. Shorter bridges favor adiabatic electron transfer with a charge-transfer emission, while longer bridges favor fast energy transfer followed by slower nonadiabatic electron transfer, resulting in dual emission in polar solvents. []
Q5: Has 2,3-Dimethoxynaphthalene been found in natural sources?
A5: Yes, 2,3-Dimethoxynaphthalene has been identified as a component of New Zealand manuka honey using gas chromatography-mass spectrometry. []
Q6: Are there any known applications of 2,3-Dimethoxynaphthalene in organic synthesis?
A6: 2,3-Dimethoxynaphthalene serves as a starting material in the synthesis of various compounds. For instance, it undergoes nitration to yield a mixture of mono-nitro, di-nitro products, and a 1,2-naphthaquinone. [] It also reacts with ethyl azidoformate through photochemical and thermal pathways, leading to substitution, addition, and insertion products. []
Q7: What is the behavior of 2,3-Dimethoxynaphthalene under sulfonation conditions?
A7: Sulfonation of 2,3-Dimethoxynaphthalene proceeds through the formation of oxonium and quinonoid intermediates. The reaction mechanism involves protonation equilibria, and the steady sulfonation rate constants are influenced by the acidity of the reaction medium. []
Q8: Are there computational studies on 2,3-Dimethoxynaphthalene?
A8: Yes, computational chemistry methods, particularly density functional theory (DFT), have been employed to investigate the vibrational spectra, molecular geometry, conformational stability, and molecular properties of 2,3-Dimethoxynaphthalene derivatives. []
Descargo de responsabilidad e información sobre productos de investigación in vitro
Tenga en cuenta que todos los artículos e información de productos presentados en BenchChem están destinados únicamente con fines informativos. Los productos disponibles para la compra en BenchChem están diseñados específicamente para estudios in vitro, que se realizan fuera de organismos vivos. Los estudios in vitro, derivados del término latino "in vidrio", involucran experimentos realizados en entornos de laboratorio controlados utilizando células o tejidos. Es importante tener en cuenta que estos productos no se clasifican como medicamentos y no han recibido la aprobación de la FDA para la prevención, tratamiento o cura de ninguna condición médica, dolencia o enfermedad. Debemos enfatizar que cualquier forma de introducción corporal de estos productos en humanos o animales está estrictamente prohibida por ley. Es esencial adherirse a estas pautas para garantizar el cumplimiento de los estándares legales y éticos en la investigación y experimentación.