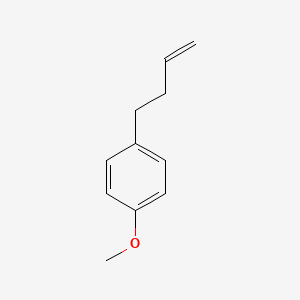
4-(4-Methoxyphenyl)-1-butene
Descripción general
Descripción
4-(4-Methoxyphenyl)-1-butene (4MPB) is a synthetic compound that has been studied for its potential applications in various scientific fields. 4MPB is a colorless liquid with a molecular weight of 204.3 g/mol and a boiling point of 132°C. It is a derivative of 1-butene and is composed of four carbon atoms, one oxygen atom, and four hydrogen atoms. It is also known as 4-methoxy-1-butene, 4-methoxy-1-butene-4-ol, and 4-methoxy-1-butene-4-ol ether.
Aplicaciones Científicas De Investigación
Synthesis of Photoluminescent 1,2-Dihydrophosphinines
4-(4-Methoxyphenyl)-1-butene is used in the synthesis of photoluminescent 1,2-dihydrophosphinines via a [4 + 2] cycloaddition . This process involves the reaction of 4-(4-Methoxyphenyl)-1-butene with other reactants to produce 1,2-dihydrophosphinines, which are compounds that emit light when excited .
Copper-Catalyzed Synthesis of Trisubstituted 1,2,4-Triazoles
This compound is also used along with an arylboronic acid and sodium azide in a copper-catalyzed, three-component synthesis of trisubstituted 1,2,4-triazoles . Triazoles are a class of organic compounds that have potential applications in medicinal chemistry due to their biological activity .
Gold (III)-Catalyzed Hydroamination of Alkynes
4-(4-Methoxyphenyl)-1-butene is used in a study of a gold (III)-catalyzed hydroamination of alkynes leading to N-vinylindoles . This process involves the addition of a nitrogen atom to an alkyne to form an indole, a heterocyclic aromatic organic compound .
Material Science
4-(4-Methoxyphenyl)-1-butene has potential applications in material science. It can be used in the synthesis of new materials with unique properties, such as high thermal stability, electrical conductivity, and resistance to chemical attack.
Organic Chemistry
In organic chemistry, 4-(4-Methoxyphenyl)-1-butene can be used as a building block for the synthesis of complex organic molecules. Its structure allows it to react with a variety of other compounds, making it a versatile tool in the synthesis of new organic compounds.
Medicine
4-(4-Methoxyphenyl)-1-butene can also be used in the field of medicine. It can be used in the synthesis of pharmaceuticals and other biologically active compounds.
Mecanismo De Acción
Target of Action
Compounds with similar structures, such as 4-methoxyamphetamine, have been found to act as potent and selective serotonin releasing agents . They bind to alpha receptors to mediate these effects .
Mode of Action
Based on the structural similarity to 4-methoxyamphetamine, it can be hypothesized that it might interact with its targets, possibly alpha receptors, leading to changes in cellular signaling .
Biochemical Pathways
For instance, 4-Phenylbutyric acid and its derivatives have been found to ameliorate unfolded proteins and suppress their aggregation, which result in protective effects against endoplasmic reticulum stress-induced neuronal cell death .
Pharmacokinetics
Compounds with similar structures have been found to exhibit strong electronic characteristics and meet the admet and drug-likeness requirements without a single instance of lipinski’s rule of five violations .
Result of Action
For instance, 4-Phenylbutyric acid and its derivatives have been found to exhibit inhibitory activity against histone deacetylases (HDACs) .
Propiedades
IUPAC Name |
1-but-3-enyl-4-methoxybenzene | |
---|---|---|
Source | PubChem | |
URL | https://pubchem.ncbi.nlm.nih.gov | |
Description | Data deposited in or computed by PubChem | |
InChI |
InChI=1S/C11H14O/c1-3-4-5-10-6-8-11(12-2)9-7-10/h3,6-9H,1,4-5H2,2H3 | |
Source | PubChem | |
URL | https://pubchem.ncbi.nlm.nih.gov | |
Description | Data deposited in or computed by PubChem | |
InChI Key |
CLTMQCGFHLPTBB-UHFFFAOYSA-N | |
Source | PubChem | |
URL | https://pubchem.ncbi.nlm.nih.gov | |
Description | Data deposited in or computed by PubChem | |
Canonical SMILES |
COC1=CC=C(C=C1)CCC=C | |
Source | PubChem | |
URL | https://pubchem.ncbi.nlm.nih.gov | |
Description | Data deposited in or computed by PubChem | |
Molecular Formula |
C11H14O | |
Source | PubChem | |
URL | https://pubchem.ncbi.nlm.nih.gov | |
Description | Data deposited in or computed by PubChem | |
DSSTOX Substance ID |
DTXSID80348952 | |
Record name | 1-(But-3-en-1-yl)-4-methoxybenzene | |
Source | EPA DSSTox | |
URL | https://comptox.epa.gov/dashboard/DTXSID80348952 | |
Description | DSSTox provides a high quality public chemistry resource for supporting improved predictive toxicology. | |
Molecular Weight |
162.23 g/mol | |
Source | PubChem | |
URL | https://pubchem.ncbi.nlm.nih.gov | |
Description | Data deposited in or computed by PubChem | |
Product Name |
4-(4-Methoxyphenyl)-1-butene | |
CAS RN |
20574-98-5 | |
Record name | 1-(But-3-en-1-yl)-4-methoxybenzene | |
Source | EPA DSSTox | |
URL | https://comptox.epa.gov/dashboard/DTXSID80348952 | |
Description | DSSTox provides a high quality public chemistry resource for supporting improved predictive toxicology. | |
Retrosynthesis Analysis
AI-Powered Synthesis Planning: Our tool employs the Template_relevance Pistachio, Template_relevance Bkms_metabolic, Template_relevance Pistachio_ringbreaker, Template_relevance Reaxys, Template_relevance Reaxys_biocatalysis model, leveraging a vast database of chemical reactions to predict feasible synthetic routes.
One-Step Synthesis Focus: Specifically designed for one-step synthesis, it provides concise and direct routes for your target compounds, streamlining the synthesis process.
Accurate Predictions: Utilizing the extensive PISTACHIO, BKMS_METABOLIC, PISTACHIO_RINGBREAKER, REAXYS, REAXYS_BIOCATALYSIS database, our tool offers high-accuracy predictions, reflecting the latest in chemical research and data.
Strategy Settings
Precursor scoring | Relevance Heuristic |
---|---|
Min. plausibility | 0.01 |
Model | Template_relevance |
Template Set | Pistachio/Bkms_metabolic/Pistachio_ringbreaker/Reaxys/Reaxys_biocatalysis |
Top-N result to add to graph | 6 |
Feasible Synthetic Routes
Descargo de responsabilidad e información sobre productos de investigación in vitro
Tenga en cuenta que todos los artículos e información de productos presentados en BenchChem están destinados únicamente con fines informativos. Los productos disponibles para la compra en BenchChem están diseñados específicamente para estudios in vitro, que se realizan fuera de organismos vivos. Los estudios in vitro, derivados del término latino "in vidrio", involucran experimentos realizados en entornos de laboratorio controlados utilizando células o tejidos. Es importante tener en cuenta que estos productos no se clasifican como medicamentos y no han recibido la aprobación de la FDA para la prevención, tratamiento o cura de ninguna condición médica, dolencia o enfermedad. Debemos enfatizar que cualquier forma de introducción corporal de estos productos en humanos o animales está estrictamente prohibida por ley. Es esencial adherirse a estas pautas para garantizar el cumplimiento de los estándares legales y éticos en la investigación y experimentación.