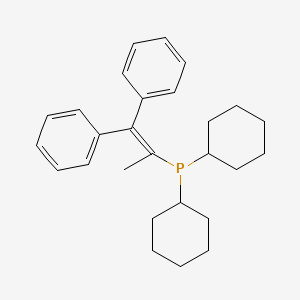
dicyclohexyl(1,1-diphenylprop-1-en-2-yl)phosphane
Descripción general
Descripción
Aplicaciones Científicas De Investigación
dicyclohexyl(1,1-diphenylprop-1-en-2-yl)phosphane is widely used in scientific research due to its effectiveness as a ligand in catalytic reactions. Its applications include:
- Chemistry : Used in the synthesis of complex organic molecules through cross-coupling reactions .
- Biology : Employed in the modification of biomolecules for research purposes .
- Medicine : Utilized in the synthesis of pharmaceutical intermediates .
- Industry : Applied in the production of fine chemicals and materials .
Mecanismo De Acción
Target of Action
Cy-vBRIDP is a type of phosphine ligand . Ligands are molecules that bind to other (usually larger) molecules, and phosphine ligands, in particular, are known for their role in palladium-catalyzed coupling reactions . The primary targets of Cy-vBRIDP are palladium species involved in these reactions .
Mode of Action
The interaction of Cy-vBRIDP with its targets involves the formation of a catalyst system. This system effectively catalyzes coupling reactions of a wide range of aryl halides with arylboronic acids, amines, and aryl ketones . The unique structure of Cy-vBRIDP, which provides high electron density on phosphorus, enhances the initial oxidative addition to the aryl halide and the final reductive elimination step of the catalytic cycle .
Biochemical Pathways
The primary biochemical pathway affected by Cy-vBRIDP is the cross-coupling reaction pathway. This pathway involves the formation of carbon-carbon and carbon-nitrogen bonds, which are key steps in the synthesis of many types of natural products, fine chemicals, pharmaceutical intermediates, and polymers . The use of Cy-vBRIDP enhances the efficiency of these reactions, particularly when using less active aryl chlorides .
Result of Action
The molecular effect of Cy-vBRIDP’s action is the facilitation of bond formation in cross-coupling reactions . On a cellular level, this can lead to the synthesis of a wide variety of compounds, depending on the specific reactants used. These compounds can have various effects, from serving as building blocks in polymer production to acting as active pharmaceutical ingredients .
Action Environment
The action of Cy-vBRIDP can be influenced by various environmental factors. For instance, its stability in air is due to its unique structure that prevents oxidation to phosphine oxides . The efficiency of the reactions it catalyzes can also be affected by factors such as temperature and the presence of other substances in the reaction mixture . .
Safety and Hazards
Direcciones Futuras
Análisis Bioquímico
Biochemical Properties
Cy-vBRIDP plays a significant role in biochemical reactions, particularly in catalysis. It is known to interact with various enzymes and proteins, facilitating reactions such as Buchwald-Hartwig cross-coupling, Heck reaction, Hiyama coupling, Negishi coupling, Sonogashira coupling, Stille coupling, and Suzuki-Miyaura coupling . The nature of these interactions involves the formation of complexes with transition metals, which then act as catalysts to accelerate the reaction rates. Cy-vBRIDP’s ability to stabilize these metal complexes makes it a valuable tool in synthetic chemistry.
Temporal Effects in Laboratory Settings
In laboratory settings, the effects of Cy-vBRIDP can change over time due to its stability and degradation. Cy-vBRIDP is generally stable when stored at temperatures between 2-8°C . Prolonged exposure to higher temperatures or reactive environments may lead to its degradation, reducing its effectiveness in biochemical reactions. Long-term studies have shown that Cy-vBRIDP can have lasting effects on cellular function, with some changes in gene expression and metabolic activity persisting even after the compound is no longer present.
Métodos De Preparación
Synthetic Routes and Reaction Conditions: dicyclohexyl(1,1-diphenylprop-1-en-2-yl)phosphane can be synthesized through the reaction of dicyclohexylphosphine with 1,1-diphenyl-1-propene under inert conditions. The reaction typically requires a base such as potassium tert-butoxide and is carried out in a solvent like tetrahydrofuran (THF) at low temperatures .
Industrial Production Methods: While specific industrial production methods for this compound are not widely documented, the compound is generally produced in laboratory settings for research purposes. The production involves standard organic synthesis techniques and purification methods such as recrystallization .
Análisis De Reacciones Químicas
Types of Reactions: dicyclohexyl(1,1-diphenylprop-1-en-2-yl)phosphane is primarily involved in various cross-coupling reactions, including:
- Buchwald-Hartwig Cross Coupling Reaction
- Heck Reaction
- Hiyama Coupling
- Negishi Coupling
- Sonogashira Coupling
- Stille Coupling
- Suzuki-Miyaura Coupling
Common Reagents and Conditions: These reactions typically involve palladium or nickel catalysts and are carried out under inert atmospheres. Common reagents include aryl halides, boronic acids, and organometallic reagents. The reactions are often performed in solvents such as toluene, THF, or dimethylformamide (DMF) at elevated temperatures .
Major Products Formed: The major products formed from these reactions are various substituted aromatic compounds, which are valuable intermediates in the synthesis of pharmaceuticals, agrochemicals, and organic materials .
Comparación Con Compuestos Similares
Similar Compounds:
- vBRIDP
- tBuXPhos
- APhos
- MorDalphos
- RockPhos
Uniqueness: dicyclohexyl(1,1-diphenylprop-1-en-2-yl)phosphane stands out due to its unique combination of steric and electronic properties, which provide high stability and reactivity in catalytic processes. Its ability to facilitate a wide range of cross-coupling reactions with high efficiency makes it a valuable ligand in organic synthesis .
Propiedades
IUPAC Name |
dicyclohexyl(1,1-diphenylprop-1-en-2-yl)phosphane | |
---|---|---|
Source | PubChem | |
URL | https://pubchem.ncbi.nlm.nih.gov | |
Description | Data deposited in or computed by PubChem | |
InChI |
InChI=1S/C27H35P/c1-22(27(23-14-6-2-7-15-23)24-16-8-3-9-17-24)28(25-18-10-4-11-19-25)26-20-12-5-13-21-26/h2-3,6-9,14-17,25-26H,4-5,10-13,18-21H2,1H3 | |
Source | PubChem | |
URL | https://pubchem.ncbi.nlm.nih.gov | |
Description | Data deposited in or computed by PubChem | |
InChI Key |
YMSBPYCREGBACF-UHFFFAOYSA-N | |
Source | PubChem | |
URL | https://pubchem.ncbi.nlm.nih.gov | |
Description | Data deposited in or computed by PubChem | |
Canonical SMILES |
CC(=C(C1=CC=CC=C1)C2=CC=CC=C2)P(C3CCCCC3)C4CCCCC4 | |
Source | PubChem | |
URL | https://pubchem.ncbi.nlm.nih.gov | |
Description | Data deposited in or computed by PubChem | |
Molecular Formula |
C27H35P | |
Source | PubChem | |
URL | https://pubchem.ncbi.nlm.nih.gov | |
Description | Data deposited in or computed by PubChem | |
DSSTOX Substance ID |
DTXSID40475778 | |
Record name | Cy-vBRIDP | |
Source | EPA DSSTox | |
URL | https://comptox.epa.gov/dashboard/DTXSID40475778 | |
Description | DSSTox provides a high quality public chemistry resource for supporting improved predictive toxicology. | |
Molecular Weight |
390.5 g/mol | |
Source | PubChem | |
URL | https://pubchem.ncbi.nlm.nih.gov | |
Description | Data deposited in or computed by PubChem | |
CAS No. |
384842-24-4 | |
Record name | Cy-vBRIDP | |
Source | EPA DSSTox | |
URL | https://comptox.epa.gov/dashboard/DTXSID40475778 | |
Description | DSSTox provides a high quality public chemistry resource for supporting improved predictive toxicology. | |
Synthesis routes and methods
Procedure details
Retrosynthesis Analysis
AI-Powered Synthesis Planning: Our tool employs the Template_relevance Pistachio, Template_relevance Bkms_metabolic, Template_relevance Pistachio_ringbreaker, Template_relevance Reaxys, Template_relevance Reaxys_biocatalysis model, leveraging a vast database of chemical reactions to predict feasible synthetic routes.
One-Step Synthesis Focus: Specifically designed for one-step synthesis, it provides concise and direct routes for your target compounds, streamlining the synthesis process.
Accurate Predictions: Utilizing the extensive PISTACHIO, BKMS_METABOLIC, PISTACHIO_RINGBREAKER, REAXYS, REAXYS_BIOCATALYSIS database, our tool offers high-accuracy predictions, reflecting the latest in chemical research and data.
Strategy Settings
Precursor scoring | Relevance Heuristic |
---|---|
Min. plausibility | 0.01 |
Model | Template_relevance |
Template Set | Pistachio/Bkms_metabolic/Pistachio_ringbreaker/Reaxys/Reaxys_biocatalysis |
Top-N result to add to graph | 6 |
Feasible Synthetic Routes
Descargo de responsabilidad e información sobre productos de investigación in vitro
Tenga en cuenta que todos los artículos e información de productos presentados en BenchChem están destinados únicamente con fines informativos. Los productos disponibles para la compra en BenchChem están diseñados específicamente para estudios in vitro, que se realizan fuera de organismos vivos. Los estudios in vitro, derivados del término latino "in vidrio", involucran experimentos realizados en entornos de laboratorio controlados utilizando células o tejidos. Es importante tener en cuenta que estos productos no se clasifican como medicamentos y no han recibido la aprobación de la FDA para la prevención, tratamiento o cura de ninguna condición médica, dolencia o enfermedad. Debemos enfatizar que cualquier forma de introducción corporal de estos productos en humanos o animales está estrictamente prohibida por ley. Es esencial adherirse a estas pautas para garantizar el cumplimiento de los estándares legales y éticos en la investigación y experimentación.