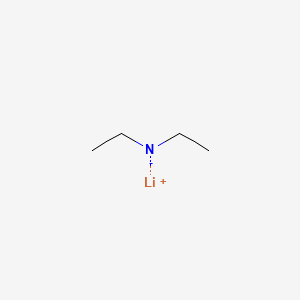
Dietilamida de litio
Descripción general
Descripción
Lithium diethylamide is an organolithium compound with the chemical formula (C₂H₅)₂NLi. It is a strong base and nucleophile, commonly used in organic synthesis for deprotonation reactions and as a reagent in various chemical transformations. The compound is characterized by its high reactivity and is often employed in the preparation of other organolithium compounds.
Aplicaciones Científicas De Investigación
- LiDEA actúa como una base poderosa en la síntesis orgánica. Se utiliza para desprotonar compuestos ácidos, facilitando la formación de complejos organoimido de metales de transición temprana .
- Los investigadores emplean LiDEA en reacciones de reordenamiento meta, como la transformación de trifenil(p-bromofenil)silano .
Química Organometálica y Síntesis
Investigación Farmacológica
Mecanismo De Acción
Target of Action
Lithium diethylamide, also known as Lysergic acid diethylamide (LSD), primarily targets the serotonin receptors in the brain . It has the highest binding affinity to serotonin receptors 5-HT 1A and 5-HT 2A located in the frontal cortex . These receptors play a crucial role in regulating mood, cognition, and perception .
Mode of Action
Lithium diethylamide interacts with its targets by binding to the serotonin receptors, particularly the 5-HT 2A receptor . This interaction leads to a variety of altered states of consciousness, facilitating the psychedelic properties of the compound . The compound’s interaction with these receptors results in changes in perception, illusory imagination, and positively experienced ego-dissolution .
Biochemical Pathways
Lithium diethylamide affects several biochemical pathways. It has been found to inhibit enzymes that have magnesium as a co-factor, such as inositol monophosphatase (IMPA) and glycogen synthase kinase-3 (GSK-3) . Its pharmacological action involves different neuronal pathways like serotonin, dopamine, glutamate, and noradrenaline circuits . The inhibition of GSK-3β is a key mechanism of lithium’s therapeutic response .
Pharmacokinetics
It is known that the compound is rapidly absorbed . It is metabolized in the liver and has a half-life of approximately 3 hours . More research is needed to fully understand the ADME properties of lithium diethylamide and their impact on its bioavailability.
Result of Action
The molecular and cellular effects of lithium diethylamide’s action are primarily observed in the central nervous system. It enhances empathy, potentially independent of its primary action on 5-HT 2A receptors . In contrast, LSD-induced oxytocin release may depend on 5-HT 2A receptor stimulation, which is consistent with the psychedelic effect of LSD . It also has effects on neurotrophic molecular pathways, providing further insights into the mechanism of lithium action .
Action Environment
Environmental factors can influence the action, efficacy, and stability of lithium diethylamide. Improper disposal or reprocessing of spent lithium batteries, which contain lithium, can lead to environmental hazards as lithium accumulates in the food chain, impacting ecosystems and human health . Therefore, proper disposal and recycling measures are crucial to offset the negative effects of used lithium batteries
Análisis Bioquímico
Biochemical Properties
Lithium diethylamide plays a crucial role in biochemical reactions due to its strong basicity. It is often used to deprotonate weak acids, facilitating the formation of carbon-carbon bonds in organic synthesis. The compound interacts with various enzymes and proteins, primarily through nucleophilic attack on electrophilic centers. For instance, lithium diethylamide can interact with carbonyl-containing biomolecules, leading to the formation of new chemical bonds. These interactions are essential for the synthesis of complex organic molecules and pharmaceuticals .
Cellular Effects
Lithium diethylamide has significant effects on various types of cells and cellular processes. It influences cell function by altering cell signaling pathways, gene expression, and cellular metabolism. The compound can induce changes in the expression of genes involved in metabolic pathways, leading to altered cellular metabolism. Additionally, lithium diethylamide can affect cell signaling pathways by interacting with key signaling molecules, thereby modulating cellular responses .
Molecular Mechanism
The molecular mechanism of lithium diethylamide involves its strong nucleophilicity and basicity. At the molecular level, lithium diethylamide exerts its effects by deprotonating weak acids and forming new chemical bonds. This process involves the interaction of lithium diethylamide with electrophilic centers in biomolecules, leading to the formation of new covalent bonds. These interactions can result in enzyme inhibition or activation, depending on the specific biomolecule involved. Additionally, lithium diethylamide can induce changes in gene expression by interacting with transcription factors and other regulatory proteins .
Temporal Effects in Laboratory Settings
In laboratory settings, the effects of lithium diethylamide can change over time. The compound is relatively stable under standard laboratory conditions, but it can degrade when exposed to moisture or air. Over time, the degradation of lithium diethylamide can lead to a decrease in its effectiveness in biochemical reactions. Long-term studies have shown that lithium diethylamide can have lasting effects on cellular function, including changes in gene expression and cellular metabolism .
Dosage Effects in Animal Models
The effects of lithium diethylamide vary with different dosages in animal models. At low doses, the compound can induce mild changes in cellular function and metabolism. At high doses, lithium diethylamide can cause toxic or adverse effects, including cellular damage and disruption of normal cellular processes. Threshold effects have been observed, where a specific dosage level leads to a significant change in the compound’s effects on cellular function .
Metabolic Pathways
Lithium diethylamide is involved in various metabolic pathways, primarily through its role as a strong base and nucleophile. The compound interacts with enzymes and cofactors involved in metabolic reactions, leading to changes in metabolic flux and metabolite levels. For example, lithium diethylamide can deprotonate intermediates in metabolic pathways, facilitating the formation of new metabolites. These interactions are crucial for the regulation of metabolic processes and the synthesis of complex organic molecules .
Transport and Distribution
Within cells and tissues, lithium diethylamide is transported and distributed through interactions with transporters and binding proteins. The compound can bind to specific transporters, facilitating its movement across cellular membranes. Additionally, lithium diethylamide can accumulate in certain cellular compartments, depending on its interactions with binding proteins. These interactions are essential for the compound’s localization and function within cells .
Subcellular Localization
The subcellular localization of lithium diethylamide is influenced by its interactions with targeting signals and post-translational modifications. The compound can be directed to specific compartments or organelles within the cell, where it exerts its biochemical effects. For instance, lithium diethylamide can be localized to the mitochondria, where it influences metabolic processes and energy production. These localization mechanisms are crucial for the compound’s activity and function within cells .
Métodos De Preparación
Synthetic Routes and Reaction Conditions: Lithium diethylamide can be synthesized through the reaction of diethylamine with lithium metal. The reaction typically takes place in an inert atmosphere to prevent the highly reactive lithium from reacting with moisture or oxygen. The general reaction is as follows:
2Li+2(C2H5)2NH→2(C2H5)2NLi+H2
The reaction is usually carried out in a hydrocarbon solvent such as hexane or cyclohexane.
Industrial Production Methods: In industrial settings, lithium diethylamide is produced in larger quantities using similar methods but with more controlled and scalable processes. The use of continuous flow reactors and automated systems ensures consistent quality and yield.
Types of Reactions:
Deprotonation: Lithium diethylamide is widely used for deprotonating weak acids, including alcohols, amines, and hydrocarbons.
Nucleophilic Addition: It can add to carbonyl compounds, forming lithium alkoxides.
Substitution Reactions: Lithium diethylamide can participate in nucleophilic substitution reactions with alkyl halides.
Common Reagents and Conditions:
Solvents: Hydrocarbon solvents like hexane and cyclohexane are commonly used.
Temperature: Reactions are typically carried out at low temperatures to control the reactivity.
Atmosphere: An inert atmosphere (e.g., nitrogen or argon) is essential to prevent unwanted side reactions.
Major Products:
Lithium Alkoxides: Formed from the reaction with carbonyl compounds.
Substituted Amines: Resulting from nucleophilic substitution reactions.
Chemistry:
Synthesis of Complex Molecules: Lithium diethylamide is used in the synthesis of pharmaceuticals, agrochemicals, and other complex organic molecules.
Polymerization: It acts as an initiator in the polymerization of certain monomers.
Biology and Medicine:
Drug Development: It is used in the synthesis of intermediates for drug development.
Bioconjugation: Lithium diethylamide can be used to modify biomolecules for research purposes.
Industry:
Material Science: It is employed in the production of advanced materials, including polymers and composites.
Catalysis: Lithium diethylamide is used as a catalyst in various industrial processes.
Comparación Con Compuestos Similares
Lithium Dimethylamide: Similar in structure but with methyl groups instead of ethyl groups.
Lithium Diisopropylamide: A more sterically hindered base, often used when selectivity is required.
Lithium Bis(trimethylsilyl)amide: A bulky base used in specific synthetic applications.
Uniqueness: Lithium diethylamide is unique due to its balance of reactivity and selectivity. It is less sterically hindered than lithium diisopropylamide, making it more reactive in certain deprotonation reactions. Compared to lithium dimethylamide, it offers a different reactivity profile due to the larger ethyl groups.
Propiedades
IUPAC Name |
lithium;diethylazanide | |
---|---|---|
Source | PubChem | |
URL | https://pubchem.ncbi.nlm.nih.gov | |
Description | Data deposited in or computed by PubChem | |
InChI |
InChI=1S/C4H10N.Li/c1-3-5-4-2;/h3-4H2,1-2H3;/q-1;+1 | |
Source | PubChem | |
URL | https://pubchem.ncbi.nlm.nih.gov | |
Description | Data deposited in or computed by PubChem | |
InChI Key |
AHNJTQYTRPXLLG-UHFFFAOYSA-N | |
Source | PubChem | |
URL | https://pubchem.ncbi.nlm.nih.gov | |
Description | Data deposited in or computed by PubChem | |
Canonical SMILES |
[Li+].CC[N-]CC | |
Source | PubChem | |
URL | https://pubchem.ncbi.nlm.nih.gov | |
Description | Data deposited in or computed by PubChem | |
Molecular Formula |
C4H10LiN | |
Source | PubChem | |
URL | https://pubchem.ncbi.nlm.nih.gov | |
Description | Data deposited in or computed by PubChem | |
DSSTOX Substance ID |
DTXSID001002077 | |
Record name | Lithium N-ethylethanaminide | |
Source | EPA DSSTox | |
URL | https://comptox.epa.gov/dashboard/DTXSID001002077 | |
Description | DSSTox provides a high quality public chemistry resource for supporting improved predictive toxicology. | |
Molecular Weight |
79.1 g/mol | |
Source | PubChem | |
URL | https://pubchem.ncbi.nlm.nih.gov | |
Description | Data deposited in or computed by PubChem | |
Physical Description |
Solid; [Sigma-Aldrich MSDS] | |
Record name | Lithium diethylamide | |
Source | Haz-Map, Information on Hazardous Chemicals and Occupational Diseases | |
URL | https://haz-map.com/Agents/21120 | |
Description | Haz-Map® is an occupational health database designed for health and safety professionals and for consumers seeking information about the adverse effects of workplace exposures to chemical and biological agents. | |
Explanation | Copyright (c) 2022 Haz-Map(R). All rights reserved. Unless otherwise indicated, all materials from Haz-Map are copyrighted by Haz-Map(R). No part of these materials, either text or image may be used for any purpose other than for personal use. Therefore, reproduction, modification, storage in a retrieval system or retransmission, in any form or by any means, electronic, mechanical or otherwise, for reasons other than personal use, is strictly prohibited without prior written permission. | |
CAS No. |
816-43-3 | |
Record name | Lithium diethylamide | |
Source | ChemIDplus | |
URL | https://pubchem.ncbi.nlm.nih.gov/substance/?source=chemidplus&sourceid=0000816433 | |
Description | ChemIDplus is a free, web search system that provides access to the structure and nomenclature authority files used for the identification of chemical substances cited in National Library of Medicine (NLM) databases, including the TOXNET system. | |
Record name | Lithium N-ethylethanaminide | |
Source | EPA DSSTox | |
URL | https://comptox.epa.gov/dashboard/DTXSID001002077 | |
Description | DSSTox provides a high quality public chemistry resource for supporting improved predictive toxicology. | |
Record name | Lithium diethylamide | |
Source | European Chemicals Agency (ECHA) | |
URL | https://echa.europa.eu/substance-information/-/substanceinfo/100.011.303 | |
Description | The European Chemicals Agency (ECHA) is an agency of the European Union which is the driving force among regulatory authorities in implementing the EU's groundbreaking chemicals legislation for the benefit of human health and the environment as well as for innovation and competitiveness. | |
Explanation | Use of the information, documents and data from the ECHA website is subject to the terms and conditions of this Legal Notice, and subject to other binding limitations provided for under applicable law, the information, documents and data made available on the ECHA website may be reproduced, distributed and/or used, totally or in part, for non-commercial purposes provided that ECHA is acknowledged as the source: "Source: European Chemicals Agency, http://echa.europa.eu/". Such acknowledgement must be included in each copy of the material. ECHA permits and encourages organisations and individuals to create links to the ECHA website under the following cumulative conditions: Links can only be made to webpages that provide a link to the Legal Notice page. | |
Synthesis routes and methods
Procedure details
Retrosynthesis Analysis
AI-Powered Synthesis Planning: Our tool employs the Template_relevance Pistachio, Template_relevance Bkms_metabolic, Template_relevance Pistachio_ringbreaker, Template_relevance Reaxys, Template_relevance Reaxys_biocatalysis model, leveraging a vast database of chemical reactions to predict feasible synthetic routes.
One-Step Synthesis Focus: Specifically designed for one-step synthesis, it provides concise and direct routes for your target compounds, streamlining the synthesis process.
Accurate Predictions: Utilizing the extensive PISTACHIO, BKMS_METABOLIC, PISTACHIO_RINGBREAKER, REAXYS, REAXYS_BIOCATALYSIS database, our tool offers high-accuracy predictions, reflecting the latest in chemical research and data.
Strategy Settings
Precursor scoring | Relevance Heuristic |
---|---|
Min. plausibility | 0.01 |
Model | Template_relevance |
Template Set | Pistachio/Bkms_metabolic/Pistachio_ringbreaker/Reaxys/Reaxys_biocatalysis |
Top-N result to add to graph | 6 |
Feasible Synthetic Routes
Q1: How does lithium diethylamide interact with organic molecules?
A1: Lithium diethylamide acts as a strong base and nucleophile due to the electron-rich nitrogen atom bearing a negative charge. It readily abstracts protons from relatively acidic compounds and adds to electrophilic centers like carbonyl groups and activated alkenes. []
Q2: What are the consequences of lithium diethylamide's basicity and nucleophilicity in organic reactions?
A2: Its strong basicity enables deprotonation of various substrates, generating reactive intermediates like enolates, carbanions, and other organolithium species. [] These intermediates can further react with electrophiles, leading to the formation of new carbon-carbon or carbon-heteroatom bonds. [, ]
Q3: What is the molecular formula and weight of lithium diethylamide?
A3: The molecular formula is C4H10LiN, and its molecular weight is 83.08 g/mol.
Q4: What spectroscopic techniques are useful for characterizing lithium diethylamide?
A4: ⁶Li and ¹⁵N NMR spectroscopy provide valuable information about the aggregation state and solvation of lithium diethylamide in solution. [] IR spectroscopy can identify characteristic vibrational modes associated with the N-H and C-H bonds.
Q5: Is lithium diethylamide sensitive to air and moisture?
A5: Yes, lithium diethylamide is highly reactive towards air and moisture. [, ] It readily decomposes upon exposure, forming lithium hydroxide and diethylamine. Therefore, handling under an inert atmosphere, like nitrogen or argon, is crucial.
Q6: What are some common applications of lithium diethylamide in organic synthesis?
A6: Lithium diethylamide is widely employed as a strong base for:
- Deprotonation of acidic protons: This generates carbanions used in alkylation, condensation, and addition reactions. [, , , , ]
- Epoxide ring-opening: This reaction affords allylic alcohols, valuable intermediates in the synthesis of complex molecules. [, ]
- Directed ortho-metalation: This reaction allows the introduction of substituents adjacent to an existing directing group on an aromatic ring. []
- Polymerization initiation: It can initiate anionic polymerizations of monomers like isoprene and styrene. [, , ]
Q7: Can you provide specific examples of reactions where lithium diethylamide acts as a catalyst?
A7: Lithium diethylamide catalyzes the addition of diethylamine to:
- Isoprene: This reaction produces 1-diethylamino-3-methyl-2-butene selectively. []
- Styrene: This reaction yields 1-diethylamino-2-phenylethane stoichiometrically. []
- meta-Divinylbenzene: This reaction allows the synthesis of new monomers with dialkylamino substituents. []
Q8: How does lithium diethylamide influence the regioselectivity of addition reactions?
A9: The regioselectivity is influenced by the steric and electronic environment of the substrate and the reaction conditions. For instance, the addition of diethylamine to isoprene catalyzed by lithium diethylamide predominantly gives the 1,4-addition product. []
Q9: How has computational chemistry been employed to study lithium diethylamide?
A9: Computational studies have been crucial in:
- Understanding the reaction mechanisms: For example, computational methods elucidated the mechanism of the reaction between lithium diethylamide and alkyl halides and sulfonates, providing insights into the competition between substitution, elimination, and sulfonation pathways. []
- Investigating the structure and aggregation state of lithium diethylamide: These studies revealed the formation of cyclic dimers, trimers, and ladder structures in solution, depending on the solvent and concentration. []
Q10: How does the structure of lithium diethylamide affect its reactivity?
A10: The bulky ethyl groups on the nitrogen atom significantly impact the reactivity of lithium diethylamide:
- Steric hindrance: The bulky groups influence the regio- and stereoselectivity of reactions by hindering the approach of the nucleophile to certain sites. [, ]
Q11: How can the stability of lithium diethylamide be enhanced?
A11: The stability of lithium diethylamide can be improved by:
Q12: What safety precautions should be taken when handling lithium diethylamide?
A12: Due to its reactivity, handling lithium diethylamide requires strict precautions:
Descargo de responsabilidad e información sobre productos de investigación in vitro
Tenga en cuenta que todos los artículos e información de productos presentados en BenchChem están destinados únicamente con fines informativos. Los productos disponibles para la compra en BenchChem están diseñados específicamente para estudios in vitro, que se realizan fuera de organismos vivos. Los estudios in vitro, derivados del término latino "in vidrio", involucran experimentos realizados en entornos de laboratorio controlados utilizando células o tejidos. Es importante tener en cuenta que estos productos no se clasifican como medicamentos y no han recibido la aprobación de la FDA para la prevención, tratamiento o cura de ninguna condición médica, dolencia o enfermedad. Debemos enfatizar que cualquier forma de introducción corporal de estos productos en humanos o animales está estrictamente prohibida por ley. Es esencial adherirse a estas pautas para garantizar el cumplimiento de los estándares legales y éticos en la investigación y experimentación.