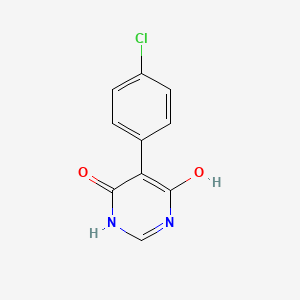
5-(4-Chlorophenyl)pyrimidine-4,6-diol
Descripción general
Descripción
5-(4-Chlorophenyl)pyrimidine-4,6-diol is a heterocyclic compound with the molecular formula C10H7ClN2O2 and a molecular weight of 222.63 g/mol . This compound features a pyrimidine ring substituted with a 4-chlorophenyl group at the 5-position and hydroxyl groups at the 4- and 6-positions. Pyrimidine derivatives are known for their diverse biological activities and are integral components of nucleic acids .
Métodos De Preparación
Synthetic Routes and Reaction Conditions
The synthesis of 5-(4-Chlorophenyl)pyrimidine-4,6-diol typically involves the condensation of 4-chlorobenzaldehyde with urea in the presence of a base, followed by cyclization and subsequent hydroxylation . The reaction conditions often include:
Base: Sodium hydroxide or potassium hydroxide
Solvent: Ethanol or methanol
Temperature: Reflux conditions (around 80-100°C)
Industrial Production Methods
Industrial production methods for this compound are not well-documented in the literature. large-scale synthesis would likely involve optimization of the above laboratory methods to improve yield and purity while minimizing costs and environmental impact.
Análisis De Reacciones Químicas
Types of Reactions
5-(4-Chlorophenyl)pyrimidine-4,6-diol can undergo various chemical reactions, including:
Oxidation: Conversion to quinones or other oxidized derivatives
Reduction: Formation of dihydropyrimidines
Substitution: Halogenation, nitration, or sulfonation at the phenyl ring
Common Reagents and Conditions
Oxidation: Potassium permanganate or chromium trioxide in acidic conditions
Reduction: Sodium borohydride or lithium aluminum hydride in anhydrous solvents
Substitution: Halogenating agents (e.g., bromine), nitrating agents (e.g., nitric acid), or sulfonating agents (e.g., sulfuric acid)
Major Products
Oxidation: Formation of 5-(4-chlorophenyl)pyrimidine-4,6-quinone
Reduction: Formation of 5-(4-chlorophenyl)dihydropyrimidine-4,6-diol
Substitution: Formation of halogenated, nitrated, or sulfonated derivatives
Aplicaciones Científicas De Investigación
5-(4-Chlorophenyl)pyrimidine-4,6-diol has several scientific research applications, including:
Mecanismo De Acción
The mechanism of action of 5-(4-Chlorophenyl)pyrimidine-4,6-diol involves its interaction with various molecular targets, including enzymes and nucleic acids. The compound can inhibit enzyme activity by binding to the active site or allosteric sites, thereby preventing substrate binding or catalysis . Additionally, its ability to intercalate into DNA can disrupt DNA replication and transcription, leading to cell cycle arrest and apoptosis .
Comparación Con Compuestos Similares
Similar Compounds
- 5-(2-Chlorophenyl)pyrimidine-4,6-diol
- 5-(4-Chlorophenyl)pyrimidine-4,6-dithiol
- 5-(4-Chlorophenyl)pyrimidine-4-thiol
Uniqueness
5-(4-Chlorophenyl)pyrimidine-4,6-diol is unique due to its specific substitution pattern, which imparts distinct chemical and biological properties. The presence of hydroxyl groups at the 4- and 6-positions enhances its ability to form hydrogen bonds, increasing its solubility and reactivity compared to similar compounds with different substituents .
Actividad Biológica
5-(4-Chlorophenyl)pyrimidine-4,6-diol is a heterocyclic compound that has garnered attention in medicinal chemistry due to its diverse biological activities. This article explores its potential applications, mechanisms of action, and relevant research findings.
Chemical Structure and Properties
The compound features a pyrimidine ring substituted with a 4-chlorophenyl group and hydroxyl groups at the 4 and 6 positions. Its molecular formula is CHClNO, with a molecular weight of 222.63 g/mol. The structural characteristics suggest potential interactions with various biological targets, which may lead to significant pharmacological effects.
Biological Activities
1. Antimicrobial Activity
Research indicates that this compound exhibits notable antimicrobial properties. In vitro studies have demonstrated its effectiveness against several bacterial strains, including both Gram-positive and Gram-negative bacteria. The minimum inhibitory concentration (MIC) values for various strains are summarized in Table 1.
Bacterial Strain | MIC (µM) |
---|---|
Staphylococcus aureus | 12 |
Escherichia coli | 18 |
Klebsiella pneumoniae | 15 |
Pseudomonas aeruginosa | 20 |
These results suggest that the compound may serve as a potential candidate for developing new antimicrobial agents.
2. Anticancer Properties
The compound has also been evaluated for its anticancer activity. Studies have shown cytotoxic effects against various cancer cell lines, including MCF-7 (breast cancer), HeLa (cervical cancer), and A549 (lung cancer). The IC50 values for these cell lines are presented in Table 2.
Cancer Cell Line | IC50 (µM) |
---|---|
MCF-7 (Breast Cancer) | 20 |
HeLa (Cervical Cancer) | 15 |
A549 (Lung Cancer) | 18 |
The mechanism of action appears to involve the induction of apoptosis through the activation of caspase pathways, highlighting its potential as a chemotherapeutic agent.
This compound interacts with various molecular targets, including enzymes and receptors involved in cellular processes:
- Enzyme Inhibition : The compound may inhibit critical enzymes necessary for pathogen survival and proliferation.
- Receptor Modulation : It can bind to specific receptors, altering their activity and potentially leading to therapeutic effects .
Case Studies
A significant study conducted by researchers at the University of Groningen focused on the compound's effects on endothelial differentiation gene (EDG-1) receptor antagonism. The findings indicated that it could play a role in preventing diseases associated with abnormal angiogenesis, such as cancer and chronic inflammatory conditions.
Future Directions
Given the promising biological activities observed, further research is warranted to explore:
- Structure-Activity Relationships (SAR) : Investigating how modifications to the structure affect biological activity.
- In Vivo Studies : Evaluating efficacy and safety in animal models.
- Pharmacological Development : Exploring its potential as a lead compound for drug development targeting specific diseases .
Q & A
Basic Research Questions
Q. What are the recommended methodologies for synthesizing 5-(4-Chlorophenyl)pyrimidine-4,6-diol with high purity and yield?
- Answer: Synthesis typically involves multi-step reactions, such as condensation of 4-chlorobenzaldehyde with urea derivatives under acidic conditions. Optimization of reaction parameters (temperature, solvent polarity, and stoichiometry) is critical. Use factorial design of experiments (DoE) to identify key variables affecting yield and purity . For example, orthogonal arrays (e.g., Taguchi methods) can minimize experimental runs while maximizing data robustness. Post-synthesis, employ column chromatography (silica gel, gradient elution) and recrystallization (ethanol/water mixtures) for purification. Validate purity via HPLC (C18 column, acetonitrile/water mobile phase) and NMR (DMSO-d6 solvent) .
Q. How can researchers resolve contradictions in spectroscopic data (e.g., NMR vs. MS) for this compound?
- Answer: Discrepancies often arise from solvent interactions, tautomeric equilibria, or impurities. For NMR:
- Compare experimental H/C spectra with computational predictions (DFT-based tools like Gaussian).
- Use 2D NMR (COSY, HSQC) to confirm proton-proton correlations and heteronuclear couplings .
For MS: Validate fragmentation patterns using high-resolution mass spectrometry (HRMS) and cross-reference with PubChem data . Always report solvent and temperature conditions to ensure reproducibility .
Q. What solvent systems are optimal for studying the solubility and stability of this compound?
- Answer: Solubility screening in DMSO, methanol, and aqueous buffers (pH 4–9) is recommended. For stability studies, use accelerated degradation tests under thermal (40–80°C) and photolytic (UV light) conditions. Monitor degradation via UV-Vis spectroscopy (λmax ~270 nm for pyrimidine derivatives). Data contradictions can be addressed using multivariate analysis (e.g., PCA) to isolate degradation pathways .
Advanced Research Questions
Q. How can computational methods enhance the design of derivatives with improved bioactivity?
- Answer: Combine density functional theory (DFT) and molecular docking to predict electronic properties (HOMO-LUMO gaps) and target binding affinities. For example:
- Use Gaussian or ORCA for DFT calculations to map electrostatic potential surfaces.
- Dock the compound into protein active sites (e.g., kinases) using AutoDock Vina or Schrödinger Suite.
Validate predictions with in vitro assays (IC50 measurements) and correlate with computational results .
Q. What advanced techniques are suitable for analyzing reaction intermediates during synthesis?
- Answer: Employ in-situ FTIR or Raman spectroscopy to monitor real-time reaction progress. For transient intermediates, use stopped-flow NMR or cryo-trapping with GC-MS. For mechanistic insights, apply kinetic isotope effects (KIE) or isotopic labeling (e.g., C tracing) . Computational reaction path searches (e.g., GRRM or AFIR methods) can also identify plausible intermediates .
Q. How can crystallographic data resolve ambiguities in molecular conformation?
- Answer: Single-crystal X-ray diffraction (SCXRD) is definitive for confirming bond angles, torsion angles, and hydrogen-bonding networks. For polymorph screening, use solvent-drop grinding or temperature-gradient crystallization. Compare experimental data with Cambridge Structural Database (CSD) entries for analogous pyrimidines. For disordered structures, refine models using SHELXL or Olex2 .
Q. Methodological Frameworks
Q. What statistical approaches are recommended for optimizing synthetic protocols?
- Answer: Use response surface methodology (RSM) with central composite design (CCD) to model non-linear relationships between variables (e.g., temperature, catalyst loading). Analyze data via ANOVA to identify significant factors (p < 0.05). For multi-objective optimization (e.g., maximizing yield while minimizing cost), apply desirability functions .
Q. How to design experiments for studying structure-activity relationships (SAR) of derivatives?
- Answer:
- Step 1: Synthesize derivatives with systematic substitutions (e.g., halogens, alkyl groups) at the 4-chlorophenyl or pyrimidine-diol positions.
- Step 2: Characterize physicochemical properties (logP, pKa) using shake-flask/HPLC methods.
- Step 3: Test bioactivity (e.g., enzyme inhibition, cytotoxicity) and apply QSAR models (e.g., CoMFA, Random Forest) to correlate structure with activity .
Q. Data Contradiction Management
Q. How to address discrepancies between computational predictions and experimental results?
- Answer: Reassess computational parameters (e.g., basis sets, solvation models). For docking, ensure protein flexibility is accounted for (e.g., ensemble docking). Experimentally, verify compound purity and assay conditions. Use Bayesian statistics to quantify uncertainty and refine models iteratively .
Q. What strategies reconcile conflicting reports on the compound’s thermal stability?
Propiedades
IUPAC Name |
5-(4-chlorophenyl)-4-hydroxy-1H-pyrimidin-6-one | |
---|---|---|
Source | PubChem | |
URL | https://pubchem.ncbi.nlm.nih.gov | |
Description | Data deposited in or computed by PubChem | |
InChI |
InChI=1S/C10H7ClN2O2/c11-7-3-1-6(2-4-7)8-9(14)12-5-13-10(8)15/h1-5H,(H2,12,13,14,15) | |
Source | PubChem | |
URL | https://pubchem.ncbi.nlm.nih.gov | |
Description | Data deposited in or computed by PubChem | |
InChI Key |
AZCIUNXJXVOJGZ-UHFFFAOYSA-N | |
Source | PubChem | |
URL | https://pubchem.ncbi.nlm.nih.gov | |
Description | Data deposited in or computed by PubChem | |
Canonical SMILES |
C1=CC(=CC=C1C2=C(N=CNC2=O)O)Cl | |
Source | PubChem | |
URL | https://pubchem.ncbi.nlm.nih.gov | |
Description | Data deposited in or computed by PubChem | |
Molecular Formula |
C10H7ClN2O2 | |
Source | PubChem | |
URL | https://pubchem.ncbi.nlm.nih.gov | |
Description | Data deposited in or computed by PubChem | |
DSSTOX Substance ID |
DTXSID00393045 | |
Record name | 5-(4-chlorophenyl)pyrimidine-4,6-diol | |
Source | EPA DSSTox | |
URL | https://comptox.epa.gov/dashboard/DTXSID00393045 | |
Description | DSSTox provides a high quality public chemistry resource for supporting improved predictive toxicology. | |
Molecular Weight |
222.63 g/mol | |
Source | PubChem | |
URL | https://pubchem.ncbi.nlm.nih.gov | |
Description | Data deposited in or computed by PubChem | |
CAS No. |
3979-80-4 | |
Record name | 5-(4-chlorophenyl)pyrimidine-4,6-diol | |
Source | EPA DSSTox | |
URL | https://comptox.epa.gov/dashboard/DTXSID00393045 | |
Description | DSSTox provides a high quality public chemistry resource for supporting improved predictive toxicology. | |
Record name | 5-(4-CHLORO-PHENYL)-PYRIMIDINE-4,6-DIOL | |
Source | European Chemicals Agency (ECHA) | |
URL | https://echa.europa.eu/information-on-chemicals | |
Description | The European Chemicals Agency (ECHA) is an agency of the European Union which is the driving force among regulatory authorities in implementing the EU's groundbreaking chemicals legislation for the benefit of human health and the environment as well as for innovation and competitiveness. | |
Explanation | Use of the information, documents and data from the ECHA website is subject to the terms and conditions of this Legal Notice, and subject to other binding limitations provided for under applicable law, the information, documents and data made available on the ECHA website may be reproduced, distributed and/or used, totally or in part, for non-commercial purposes provided that ECHA is acknowledged as the source: "Source: European Chemicals Agency, http://echa.europa.eu/". Such acknowledgement must be included in each copy of the material. ECHA permits and encourages organisations and individuals to create links to the ECHA website under the following cumulative conditions: Links can only be made to webpages that provide a link to the Legal Notice page. | |
Retrosynthesis Analysis
AI-Powered Synthesis Planning: Our tool employs the Template_relevance Pistachio, Template_relevance Bkms_metabolic, Template_relevance Pistachio_ringbreaker, Template_relevance Reaxys, Template_relevance Reaxys_biocatalysis model, leveraging a vast database of chemical reactions to predict feasible synthetic routes.
One-Step Synthesis Focus: Specifically designed for one-step synthesis, it provides concise and direct routes for your target compounds, streamlining the synthesis process.
Accurate Predictions: Utilizing the extensive PISTACHIO, BKMS_METABOLIC, PISTACHIO_RINGBREAKER, REAXYS, REAXYS_BIOCATALYSIS database, our tool offers high-accuracy predictions, reflecting the latest in chemical research and data.
Strategy Settings
Precursor scoring | Relevance Heuristic |
---|---|
Min. plausibility | 0.01 |
Model | Template_relevance |
Template Set | Pistachio/Bkms_metabolic/Pistachio_ringbreaker/Reaxys/Reaxys_biocatalysis |
Top-N result to add to graph | 6 |
Feasible Synthetic Routes
Descargo de responsabilidad e información sobre productos de investigación in vitro
Tenga en cuenta que todos los artículos e información de productos presentados en BenchChem están destinados únicamente con fines informativos. Los productos disponibles para la compra en BenchChem están diseñados específicamente para estudios in vitro, que se realizan fuera de organismos vivos. Los estudios in vitro, derivados del término latino "in vidrio", involucran experimentos realizados en entornos de laboratorio controlados utilizando células o tejidos. Es importante tener en cuenta que estos productos no se clasifican como medicamentos y no han recibido la aprobación de la FDA para la prevención, tratamiento o cura de ninguna condición médica, dolencia o enfermedad. Debemos enfatizar que cualquier forma de introducción corporal de estos productos en humanos o animales está estrictamente prohibida por ley. Es esencial adherirse a estas pautas para garantizar el cumplimiento de los estándares legales y éticos en la investigación y experimentación.